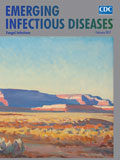
Volume 23, Number 2—February 2017
Dispatch
Determination of Elizabethkingia Diversity by MALDI-TOF Mass Spectrometry and Whole-Genome Sequencing
On This Page
Abstract
In a hospital-acquired infection with multidrug-resistant Elizabethkingia, matrix-assisted laser desorption/ionization time-of-flight mass spectrometry and 16S rRNA gene analysis identified the pathogen as Elizabethkingia miricola. Whole-genome sequencing, genus-level core genome analysis, and in silico DNA-DNA hybridization of 35 Elizabethkingia strains indicated that the species taxonomy should be further explored.
Elizabethkingia are gram-negative, nonmotile, nonspore-forming rods that are rarely associated with human disease. E. meningoseptica is the most recognized clinically, mainly causing hospital-acquired infections, including sepsis in immunocompromised patients and meningitis in neonates (1). These bacteria are intrinsically resistant to β-lactam antimicrobial drugs, including second- and third-generation cephalosporins and carbapenems, because they naturally carry an Ambler class A serine β-lactamase gene blaCME, which encodes a penicillin- and cephalosporin-hydrolyzing enzyme (2), and 2 Ambler class B metallo-β-lactamase genes, blaB and blaGOB, which encode carbapenem-hydrolyzing enzymes (3).
The species E. anopheles and E. miricola are increasingly being isolated and cause similar infections to E. meningoseptica (4,5). E. miricola was discovered in condensed water collected onboard the Russian space station Mir in 2003 (6), and E. anopheles was isolated from the midgut of the Anopheles gambiae mosquito in 2011 (7). We report infection with a carbapenem-resistant Elizabethkingia species in a patient in Copenhagen, Denmark in September 2015; we identified the bacterium as E. miricola by using MALDI-TOF (matrix-assisted laser desorption/ionization time-of-flight) mass spectrometry. We further studied the isolate using whole-genome sequencing (WGS) and compared it with other available Elizabethkingia genomes.
The patient was a 64-year-old man with recurrent erysipelas of both legs. He was initially hospitalized with erysipelas in August 2015 and successfully treated with penicillin and dicloxacillin. On readmission in September 2015, he was again treated with intravenous penicillin and dicloxacillin. Six days later, the patient felt increased pain, and his knee became warm and sore. These findings were interpreted to be symptoms of reactive arthritis caused by a urinary tract infection, and his drug treatment was changed to cefuroxime. The following day, a cloudy synovial fluid was aspired from his knee, so ciprofloxacin was added to his drug regimen. Because of aggravating symptoms and increasing C-reactive protein levels, the patient’s treatment was then changed to piperacillin with tazobactam, after which his symptoms improved. The patient was discharged with oral dicloxacillin and ciprofloxacin, and 4 weeks later he was well.
An overnight culture of the synovial fluid from the patient’s knee grew multidrug-resistant, gram-negative, nonmotile rods. MALDI-TOF (MALDI Biotyper 3.1, Bruker Daltonics Microflex LT, database MBT DB-5627) mass spectrometry identified the colonies as E. miricola (score 2.105). Antimicrobial susceptibility testing showed that the isolate was susceptible to ciprofloxacin (MIC 0.25 mg/L) and co-trimoxazole (MIC 0.125 mg/L); intermediately susceptible to piperacillin/tazobactam (MIC 16 mg/L), amoxicillin/clavulanic acid (MIC 8 mg/L), and tigecycline (MIC 0.5 mg/L); and resistant to all other drugs tested (Table). The imipenem/imipenem-EDTA Etest (bioMérieux, Marcy l’Etoile, France) for detection of metallo-β-lactamase was positive.
To confirm the species identity and identify the antimicrobial resistance genes, we performed WGS of the isolate with the Illumina MiSeq platform producing 2 × 150-bp paired-end reads by using the Nextera XT library preparation kit (Illumina Denmark ApS, Copenhagen, Denmark). Reads were assembled by using SPAdes, which produced a 4.13-Mbp genome termed HvH-WGS333 (BioProject PRJNA335686, BioSample SAMN05450241). We performed annotation of the genome using Prokka (8) and used ARG-ANNOT (9) to identify 2 metallo-β-lactamase genes, namely blaB-4 and blaGOB-13, and an extended-spectrum β-lactamase gene sharing 90.6% nucleotide identity with blaCME-1. The isolate was identified by EzTaxon (10) as E. miricola on the basis of its 16S rRNA gene with 99.69% similarity. However, single-nucleotide variant (SNV) analysis by read alignment with Bowtie2 and SNV-calling with SAMtools comparing HvH-WGS333 and the GTC 862 E. miricola strain (accession no. NZ_LSGQ00000000) yielded ≈78,000 SNVs. Furthermore, analyzing the coverage breadth by using BEDTools revealed that this isolate shared 80.5% of this E. miricola genome.
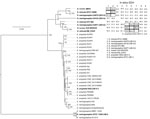
Figure. Phylogenetic tree of Elizabethkingia isolate from a patient with hospital-acquired septic arthritis in Copenhagen, Denmark 2015 compared with reference strains and in silico DNA-DNA hybridization (DDH). Tree was produced by using...
Because the isolate from this patient was not closely related to the E. miricola type strain, we performed a genus-level core genome analysis of 35 publicly deposited Elizabethkingia strains from GenBank to identify a more closely related isolate. Each genome was annotated (8) and the core genome was approximated by ROARY (11), yielding a 419,813-bp core genome of 426 genes. We constructed a phylogenetic tree of the core genome using RAxML (12) with 100 bootstrapping replicates (Figure). The ATCC 13253 E. meningoseptica strain did not cluster with the other E. meningoseptica strains and was the most distantly related Elizabethkingia sp. analyzed. Before WGS was commonly used, high levels of divergence were reported among E. meningoseptica (formerly Flavobacterium meningosepticum) isolates, with 2 groups detected, Ursing and Bruun (UB) I and UBII (13), with ATCC 13253 belonging to UBI. Our HvH-WGS333 isolate was most closely related to EM_CHUV, an endotracheal isolate submitted as E. miricola; these 2 isolates are next most closely related to G4071, which belongs to the UBII:2 (13), and then to GTC 862 E. miricola. E. miricola ATCC 33958 is most closely related to G4075, a clinical blood culture E. meningoseptica in the UBII:3 group. Strains belonging to groups UBI and UBII are phenotypically very similar, explaining why DNA-DNA hybridization (DDH) was previously used to distinguish these groups (14).
Additionally, we performed in silico DDH (15) to other sequenced Elizabethkingia isolates to determine whether our isolate could be defined as the same species (i.e., having a DDH value >70%). The in silico DDH value is calculated by summing up the identities in matches between the genome sequences and dividing by the total match hit lengths. HvH-WGS333 only had an in silico DDH >70% with EM_CHUV and G4071, which clustered most closely together in the tree but was also closely related (67.1%) to strain GTC 862 (Figure). A previous DDH study investigating the Elizabethkingia genus found DDH values at 70°C of 52% between UBII:2 and UBII:3, 45% between UBII:2 and UBII:4, and 36% between UBII:3 and UBII:4 (14), in agreement with our in silico DDH results (Figure). Our analyses support the identification of the 3 UBII subgroups 2, 3, and 4 as being 3 different Elizabethkingia species, with UBII:2 being the closest related to the E. miricola type strain.
The wide adoption of MALDI-TOF mass spectrometry for bacterial identification is likely to increase the detection of infections caused by Elizabethkingia, and thus the number of infection reports. However, the applied MALDI-TOF database contained only 3 E. meningoseptica and 1 E. miricola isolates at the time of our study. Identification on the basis of the 16S rRNA gene corroborated our MALDI-TOF mass spectrometry identification of E. miricola. When exploring this further using WGS, we discovered our isolate shared 80.5% of the GTC 862 E. miricola genome and the in silico DDH value was 67.1%, just below the 70% species threshold. It thus appears that our isolate, along with EM_CHUV and G4071, is closely related to the E. miricola species and that the taxonomic position of the UBII:3 and UBI:4 strains should be reconsidered. In light of our WGS analysis demonstrating considerable Elizabethkingia genetic diversity, we suggest that the MALDI-TOF database needs to be updated and the nomenclature needs to be re-examined. WGS is proving to be a valuable tool for the correct identification of new species and detailed characterization of multidrug-resistant bacteria.
Dr. Eriksen is training for specialization in Clinical Microbiology and is affiliated with the Department of Clinical Microbiology, Copenhagen University Hospital, Hvidovre, Denmark. Her primary research interests are epidemiology, bacteriology, and whole genome sequencing.
References
- Jean SS, Lee WS, Chen FL, Ou TY, Hsueh PR. Elizabethkingia meningoseptica: an important emerging pathogen causing healthcare-associated infections. J Hosp Infect. 2014;86:244–9. DOIPubMed
- Rossolini GM, Franceschini N, Lauretti L, Caravelli B, Riccio ML, Galleni M, et al. Cloning of a Chryseobacterium (Flavobacterium) meningosepticum chromosomal gene (blaA(CME)) encoding an extended-spectrum class A beta-lactamase related to the Bacteroides cephalosporinases and the VEB-1 and PER beta-lactamases. Antimicrob Agents Chemother. 1999;43:2193–9.PubMed
- Bellais S, Aubert D, Naas T, Nordmann P. Molecular and biochemical heterogeneity of class B carbapenem-hydrolyzing beta-lactamases in Chryseobacterium meningosepticum. Antimicrob Agents Chemother. 2000;44:1878–86. DOIPubMed
- Lau SK, Chow WN, Foo CH, Curreem SO, Lo GC, Teng JL, et al. Elizabethkingia anophelis bacteremia is associated with clinically significant infections and high mortality. Sci Rep. 2016;6:26045. DOIPubMed
- Rossati A, Kroumova V, Bargiacchi O, Brustia D, Luigi Garavelli P. Elizabethkingia miricola bacteriemia in a young woman with acute alcoholic pancreatitis. Presse Med. 2015;44:1071–2. DOIPubMed
- Li Y, Kawamura Y, Fujiwara N, Naka T, Liu H, Huang X, et al. Chryseobacterium miricola sp. nov., a novel species isolated from condensation water of space station Mir. Syst Appl Microbiol. 2003;26:523–8. DOIPubMed
- Kämpfer P, Matthews H, Glaeser SP, Martin K, Lodders N, Faye I. Elizabethkingia anophelis sp. nov., isolated from the midgut of the mosquito Anopheles gambiae. Int J Syst Evol Microbiol. 2011;61:2670–5. DOIPubMed
- Seemann T. Prokka: rapid prokaryotic genome annotation. Bioinformatics. 2014;30:2068–9. DOIPubMed
- Gupta SK, Padmanabhan BR, Diene SM, Lopez-Rojas R, Kempf M, Landraud L, et al. ARG-ANNOT, a new bioinformatic tool to discover antibiotic resistance genes in bacterial genomes. Antimicrob Agents Chemother. 2014;58:212–20. DOIPubMed
- Kim OS, Cho YJ, Lee K, Yoon SH, Kim M, Na H, et al. Introducing EzTaxon-e: a prokaryotic 16S rRNA gene sequence database with phylotypes that represent uncultured species. Int J Syst Evol Microbiol. 2012;62:716–21. DOIPubMed
- Page AJ, Cummins CA, Hunt M, Wong VK, Reuter S, Holden MT, et al. Roary: rapid large-scale prokaryote pan genome analysis. Bioinformatics. 2015;31:3691–3. DOIPubMed
- Stamatakis A. RAxML version 8: a tool for phylogenetic analysis and post-analysis of large phylogenies. Bioinformatics. 2014;30:1312–3. DOIPubMed
- Holmes B, Steigerwalt AG, Nicholson AC. DNA-DNA hybridization study of strains of Chryseobacterium, Elizabethkingia and Empedobacter and of other usually indole-producing non-fermenters of CDC groups IIc, IIe, IIh and IIi, mostly from human clinical sources, and proposals of Chryseobacterium bernardetii sp. nov., Chryseobacterium carnis sp. nov., Chryseobacterium lactis sp. nov., Chryseobacterium nakagawai sp. nov. and Chryseobacterium taklimakanense comb. nov. Int J Syst Evol Microbiol. 2013;63:4639–62. DOIPubMed
- Bruun B, Ursing J. Phenotypic characterization of Flavobacterium meningosepticum strains identified by DNA-DNA hybridization. Acta Pathol Microbiol Immunol Scand B. 1987;95:41–7.PubMed
- Meier-Kolthoff JP, Auch AF, Klenk HP, Göker M. Genome sequence-based species delimitation with confidence intervals and improved distance functions. BMC Bioinformatics. 2013;14:60. DOIPubMed
No hay comentarios:
Publicar un comentario