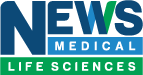
The Human Mini Heart-in-a-Jar That Could One Day Replace Animal Testing
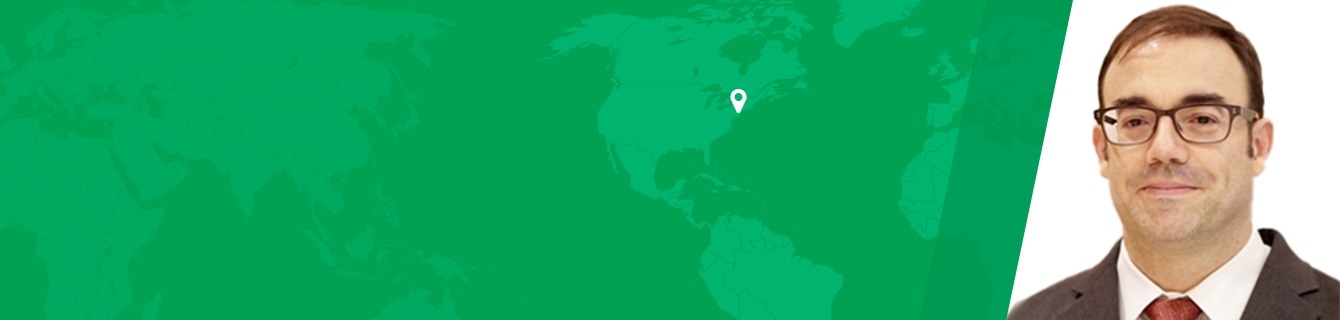
An interview with Dr. Kevin Costa, discussing the miniaturized heart-in-a-jar model developed by Novoheart, and its applications in personalized medicine.
What is the mini heart-in-a-jar?
The mini-heart is a 3D engineered, living miniature human heart chamber designed to mimic the pumping function of the human heart. It's a single-chamber organoid model that’s about a centimeter in diameter, representing the ventricle which is the key chamber in the heart for pumping blood through the body, and also the one that’s most susceptible to arrhythmias and diseases that adversely affect heart function.
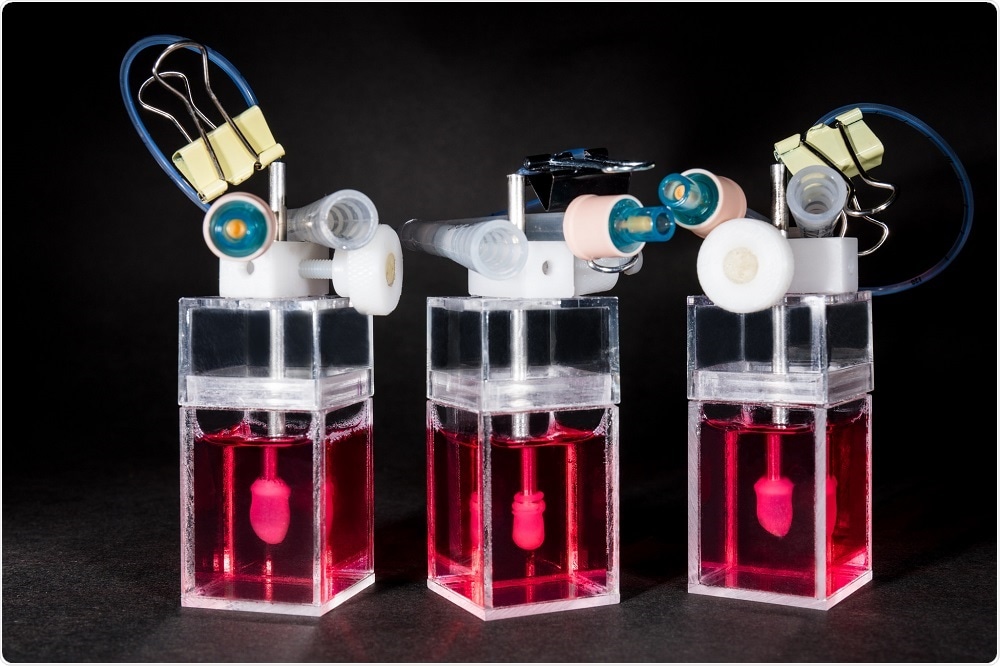
We start with human pluripotent stem cells and differentiate these into ventricular-specific cardiomyocytes, which are characteristic of the ventricles. We then embed these cells into a hydrogel matrix made of collagen and other supporting factors that are poured into a custom developed mold. The cells attach to the matrix over a couple of days and grow into a hollow, spherical shape, following the mold.
With time in culture, the cells compact the collagen around the mold and become organized into a three-dimensional tissue. Part of the mold is similar to a rubber balloon – we deflate this to leave a hollow organoid chamber that pumps and beats like a miniature human heart.
We can control the heart rate using an electrical pacemaker, meaning we can simulate the heart under different conditions such as exercise, stress or sleep, and monitor the mini-heart using sensors to measure things like pressure and volume.
This allows us to make measurements that cardiologists are interested in, such as stroke volume, ejection fraction, and cardiac output. By combining the pressure and volume measurements, we can generate pressure/volume (PV) loops, which describe the pumping efficiency of the heart. This capability is rather unique for an in vitro model of the heart.
How easy is it to keep these mini-hearts alive?
One of the other aspects that we’re focused on is developing the technology to support the biologics. It's not just about engineering and innovating tissues but also the development of bioreactors. These are machines which are used to grow and monitor the mini-heart.
We designed these bioreactors so that there is minimal user manipulation of the mini-heart, with the idea being that it will grow on its own. There’s a little bit of intervention needed to withdraw the ‘balloon’, but the measurements are pretty hands-off. This helps to maximize throughput and minimize variability between users.
We’ve also developed software and analytics that help cardiologists and scientists discover the full capabilities of the mini-heart. They’re pretty sophisticated models and can be used to generate very complicated datasets. It would be a shame to just analyze the amplitude of the pressure wave, for example.
If you start integrating pressure measurements with volume and flow, you can start to build up a more complex picture of the organoid function, which contains important information about how the system responds to drugs, or how it reproduces aspects of a particular heart disease.
To fully utilize the valuable datasets we get from studying these mini-hearts, we’ve now started to integrate machine learning technologies to help analyze all of this data in an unbiased way. For example, the machine can assess whether a drug is having a positive or a negative effect based on how the chamber is working, in a highly efficient and effective manner.
This is only the basics of what machine learning can do, in reality, there are a lot more subtleties. Just one of the many possibilities would be to develop algorithms that can be trained to automatically classify new compounds into pre-existing drug categories based on how they work. They can identify whether a drug is a sodium channel blocker or calcium channel blocker, for example.
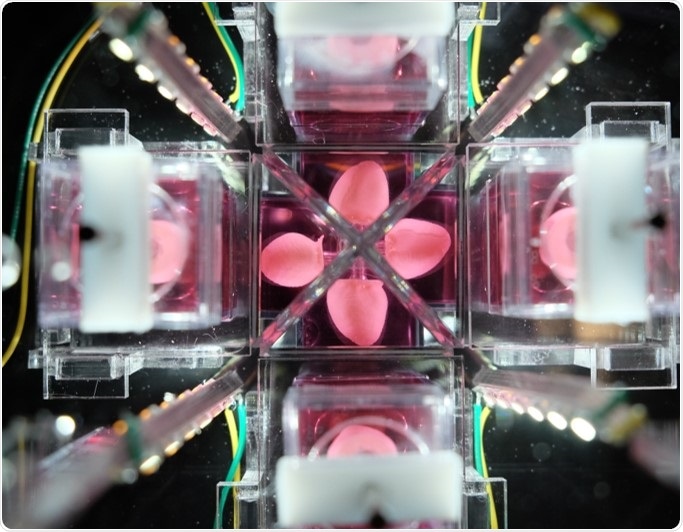
How long does it take to create one mini-heart?
Once we have the stem cells, it takes a minimum of two weeks to turn those into beating cardiomyocytes. We then make the organoid chamber by placing the cells into the custom mold. The next day, we add some additional media to help culture the cells, and after 24 hours, we remove the outside of the mold, giving the cells full access to the culture media.
Within a couple of days, the cells will start automatically beating and after 7-10 days, we remove the silicone balloon from inside the structure and are left with a hollow, autonomous beating human mini-heart chamber.
An exciting thing about this technology is that it uses induced pluripotent stem (iPS) cells. This means that if you're interested in looking at how a drug might have toxic effects on the heart, you can test it on human cells immediately, with limited ethical implications.
We can also test a drug that is expected to cure heart disease on a model that’s been made with stem cells from a patient with the disease of interest. This offers a powerful tool in drug discovery, as it can give you information about both drug efficacy using diseased mini-hearts and cardiotoxicity using healthy mini-hearts.
What makes the heart-in-a-jar so revolutionary compared to previous tools in drug development?
Traditional tools used in drug development are single-cell cultures and small animal models, which have been tested for decades. Unfortunately, history tells us that those models are not especially predictive of how a drug will ultimately behave when it's delivered to human patients.
We're exceptionally good at curing heart disease and cancer in mice and rats, but a lot of those same therapies are not effective or even toxic when delivered to patients.
Novoheart’s mini-heart technology offers a human-based system using human heart cells. It’s all part of the stem cell revolution that occurred when iPS cells were developed.
We now have access to human tissues that we just didn’t have access to in the past. Human hearts are almost impossible to come by as there are so many critically ill patients in need of a transplant. It would be unethical to ship needed organs off to a company for drug testing.
When we present this to researchers, they recognize that it is really quite unique and potentially disruptive, in the sense that for the first time, you can actually have a miniature human heart in your laboratory for several weeks.
It's not something that's going to die after a couple of hours, and it gives you human-specific measures that should better predict how therapies will behave in actual patients. Ultimately this will make therapies safer and more effective for patients, while also reducing the wasted investment of resources required to develop a drug that is destined to fail in the clinic.
Could the model be used routinely in drug development?
Yes, that's what we are striving for. A key question we are working to answer right now is the most appropriate time in the development pipeline to implement the mini-hearts.
Of course, one idea is the earlier, the better. If something's not going to work, why bother spending time developing it?
On the other hand, pharmaceutical companies have libraries containing thousands of compounds that they're interested in screening, so developing a mini-heart for each one isn’t very practical with such a system. For early stage screening, you need an assay with much higher throughput.
We imagine the right time point for using Novoheart technology is mid-stage in the drug development pipeline, when you've already identified a few lead compounds, maybe a couple of dozen or so, that you are starting to seriously invest in and think about moving to animal studies. Before doing this, you would use the organoid chambers as a screen to inform how to move forward.
For Novoheart, mini-hearts are a first step. We're heart specialists so it made sense for us to start there. Moreover, cardiotoxicity is one of the key reasons drugs fail in clinical trials. Ultimately we want to integrate heart organoids with liver organoids and lung organoids etc., to build up a whole system.
The bioreactor technology development has been designed with that plan in mind. We expect that at some stage we’ll have several organoids all interacting and being monitored by the same bioreactor, and hope that this might eventually be able to replace animal models altogether.
What’s next for Novoheart?
More immediately than a mini “human-in-a-jar”, I think validation is still key and one of our biggest priorities is to continue to generate data that showcase where these organoids shine and where they give us clear advantages compared to other systems. We also want to discover and overcome any limitations as well.
One of the challenges with using stem cells as the starting material is that the heart cells you derive from those tend to be immature. You can imagine that if you're studying embryonic cells, the first differentiation that you get is embryonic heart and then it becomes more like a newborn heart muscle and then eventually turns into an adult heart muscle. In life, that takes a couple of decades.
We think that in a laboratory setting, we can accelerate that process and drive maturation much more quickly, enabling us to study the effects of a drug at each stage of development.
Recently, we discovered that if we compare the maturation signatures of cardiomyocytes in a 2D tissue culture versus a cardiac organoid chamber, they mature faster in the organoid chamber in response to a more “heart-like” system. They're more predictive of how an adult heart would respond to some different drugs that we've been testing.
One of the other things that we're continuing to do is push the limits on the organoids and understand how they behave under more varied systems.
We're starting to increase the kinds of disease models that we can represent to include more acquired heart diseases, which are much more prevalent in the human population.
We’re also increasing the throughput of our systems so that larger populations of these organoids can be tested at the same time, which should make them more useful in selecting drugs for clinical trials.
We are also constantly continuing to improve our data analysis and machine learning aspects. Altogether, I think that's what ultimately distinguishes the kind of data we obtain and what we can do with that data versus a number of other competitors, by maximizing the information and the predictive ability of Novoheart’s MyHeartTM Platform of human cardiac-specific assays.
Where can readers find more information?
- Bioengineering an electro-mechanically functional miniature ventricular heart chamber from human pluripotent stem cells. Biomaterials. 2018.
- Novoheart website.
About Dr. Kevin Costa
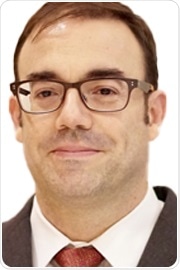
Kevin helped develop one of the first engineered cardiac tissue systems as well as several cardiac tissue engineering technologies. Since 2009, he has been working with Professor Ronald Li to translate such systems into human cells.
The work has received research funding from the Whitaker Foundation, the National Science Foundation (NSF) and the National Institutes of Health (NIH; NHLBI, NIBIB, and NIGMS). Kevin was also a recipient of the prestigious Faculty Early Career Development (CAREER) Award from the NSF.
About Novoheart
Novoheart is a global stem cell biotechnology company pioneering an array of next-generation human heart tissue prototypes. It is the first company in the world to have engineered miniature living human heart pumps that can revolutionize drug discovery, helping to save time and money for developing new therapeutics
No hay comentarios:
Publicar un comentario