Volume 25, Number 1—January 2019
Research
Multiple Introductions of Domestic Feline Leukemia Virus in Endangered Florida Panthers1
On This Page
Abstract
The endangered Florida panther (Puma concolor coryi) had an outbreak of infection with feline leukemia virus (FeLV) in the early 2000s that resulted in the deaths of 3 animals. A vaccination campaign was instituted during 2003–2007 and no additional cases were recorded until 2010. During 2010–2016, six additional FeLV cases were documented. We characterized FeLV genomes isolated from Florida panthers from both outbreaks and compared them with full-length genomes of FeLVs isolated from contemporary Florida domestic cats. Phylogenetic analyses identified >2 circulating FeLV strains in panthers, which represent separate introductions from domestic cats. The original FeLV virus outbreak strain is either still circulating or another domestic cat transmission event has occurred with a closely related variant. We also report a case of a cross-species transmission event of an oncogenic FeLV recombinant (FeLV-B). Evidence of multiple FeLV strains and detection of FeLV-B indicate Florida panthers are at high risk for FeLV infection.
Feline leukemia virus (FeLV) is a common pathogenic infectious disease responsible for high mortality rates for domestic cats, particularly before development of effective vaccines in the 1980s (1). Subgroup FeLV-A, which is replication competent and horizontally transmissible, is responsible for most infections (1,2). Other FeLV subgroups (B, C, D, E, and T) arose after recombination or through mutation (3). FeLV causes immunosuppressive, neoplastic, and hematopoietic disorders that correlate with FeLV subgroups (4–6). Virulent FeLV-B, the most common novel variant, arose after recombination between FeLV-A and endogenous FeLV (enFeLV) present in the domestic cat genome and resulted in altered cellular tropism (1,7–10). Horizontal transmission of FeLV-B is rare in domestic cats and is believed to require cotransmission with FeLV-A as a helper virus because of its replication-defective nature (11,12).
FeLV prevalence in domestic cats is variable (prevalence range 3%–18%) (13–16). FeLV has the capacity to infect nondomestic species including, but not limited to, jaguars, bobcats, the critically endangered Iberian lynx, and pumas, most notably the endangered puma subspecies, the Florida panther (Puma concolor coryi) (17–21). In all non-Felis spp. FeLV cases, the source was believed to be domestic cats, which serve as the dominant primary host. The genus Felis is the only taxon known to harbor enFeLV (22). The presence of FeLV genetic sequences in the germline results in recombination between exogenous FeLV and FeLV-A during domestic cat infections and in emergence of more deleterious subgroups (i.e., FeLV-B) that are not considered to be replication-competent in the absence of co-infection with FeLV-A (12). It is assumed that felids belonging to genera other than Felis are only infected with FeLV-A because they do not harbor enFeLV genomes.
Outbreaks of infection with FeLV have caused concern in endangered felids that have population bottlenecks because the species might be more vulnerable to infection because of reduced genetic diversity. For example, 21% of Iberian lynx sampled during 2003–2007 were FeLV positive; 6 died from FeLV-related disease (23). During 2001–2004, an outbreak of infection with FeLV was documented in Florida panthers (19). Ten Florida panthers were FeLV PCR positive, and 5 of these panthers were also determined to be antigen ELISA positive. Three deaths were attributed to FeLV-related disease (19,24). Phylogenetic analysis of a region of the FeLV env gene during this outbreak indicated a single circulating FeLV strain, likely following introduction of the virus from a domestic cat (24). The Florida Fish and Wildlife Conservation Commission (FFWCC; Tallahassee, FL, USA) attempted to contain the FeLV outbreak by implementing a vaccination campaign spanning 2003–2007 (19).
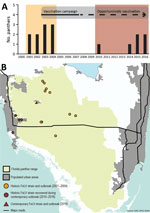
Figure 1. Temporally and spatially distinct FeLV cases in endangered Florida panthers, Florida, USA. A) Incidence of FeLV in live caught and necropsied Florida panthers. Recaptured panthers are not represented. Different colors indicate...
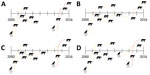
Figure 2. Hypotheses for feline leukemia virus outbreaks in Florida panthers, Florida, USA. A) Secondary infection; B) persistent transmission of 1 virus strain; C) combination of the 2 scenarios; D) multiple infections. Transmission...
During August 2004–November 2010, ≈125 live-captured or necropsied panthers were tested for FeLV, and no additional cases were detected (FFWCC, unpub. data). However, since December 2010, a total of 6 Florida panthers found dead were positive for FeLV antigenemia. These cases are separated in both time and space from the 2001–2004 outbreak (Figure 1). Four likely scenarios exist to explain the epidemic curve. First, absence of FeLV (2004–2010) might resulted from complete eradication of the first outbreak virus, followed by introduction of another strain from domestic cats (Figure 2, panel A). Second, contemporary cases might have arisen from infection that persisted but was unrecognized for 6 years (Figure 2, panel B). Third, new cases may have resulted from a combination of scenarios 1 and 2 (Figure 2, panel C). Fourth, new cases might be explained by introduction of multiple strains (Figure 2, panel D).
In this study, we examined the genetic relatedness between new FeLV isolates and cases described before 2004 with 3 aims. First, we attempted to establish whether recent cases represent a new outbreak or continuation of the prior infection. Second, we sought to determine the genetic relationship between Florida panther and Florida domestic cat FeLV strains. Third, we sought to gain insights for pathogen–host interactions to better inform management practices and reduce risk for FeLV spillover from domestic cats to endangered felid populations.
Sample Collection and Processing
FFWCC routinely screens samples collected from Florida panthers for FeLV antigenemia and FIV antibodies by using commercially available test kits (SNAP Combo FeLV/FIV test; IDEXX Laboratories, https://www.idexx.com/en). Surveillance includes animals reported dead or those live captured as part of ongoing health monitoring and population management efforts. We calculated 95% CIs of prevalence for outbreak periods and the intervening quiescent period by using the Wilson score method without continuity correction (25).
During 2010–2016, six (2.8%) of the 214 Florida panthers (P. concolor coryi UCF149, UCFP 228, FP231, UCFP241, UCFP269, and UCFP275) (Table 1) reported dead to the FFWCC were positive for FeLV antigen during standard postmortem testing on heart, chest, or venous blood collected at necropsy. Lymphoid tissues from FeLV-positive and FeLV-negative controls (i.e., bone marrow, lymph node, spleen, and thymus) in addition to a fibroblast tissue culture from 1 panther (UCFP241R1) were harvested in Florida, stored at −80°C, and shipped to the Feline Retrovirus Research Laboratory at Colorado State University for additional testing.
We isolated genomic DNA from tissues by using bead-beater disruption and phenol-chloroform extraction adapted from Fan and Gulley (26). We extracted ≈100 mg of each tissue by using 1.4-mm ceramic spheres in a Fastprep-24 tissue homogenizer (MP Biomedicals Inc., https://www.mpbio.com). Sodium dodecyl sulfate (3 mol/L) was added to the tissue homogenates at a final concentration of 10% and incubated at 37°C overnight. Cell lysates were washed twice with phenol-chloroform. Extracted DNA was concentrated by ethanol precipitation, pelleted, dried, and resuspended in TE buffer.
In addition to tissues from the contemporary outbreak, we analyzed DNA from 3 historically infected (2002–2004; FFWCC) FeLV-infected Florida panthers (FP115, FP122, and FP132), and 4 domestic cats from Florida (x1608, x1613, x2653, and x2655) collected during 2008–2018 for FeLV genomes (Table 1). Florida panther samples were obtained as described (19). Domestic cat samples were remnants of archival samples from animals brought to shelters (27) or provided by veterinary clinics to FFWCC. An additional FeLV-positive DNA blood sample obtained from a domestic cat (x2512) was included for analysis in this study (Table 1) (28).
FeLV Genome Recovery and Analyses
We sequenced full FeLV proviral genomes (8,448 bp) from 4 domestic cats (1 from Maryland and 3 from Florida), 4 of 6 contemporary Florida panthers (2010–2016), and 1 historic Florida panther (2001–2004) (Table 1). We generated two 5-kb fragments spanning the ≈8.4-kb genome, which were overlapped 1.5 kb. PCRs contained 500 nmol/L of each primer, HiFi Kapa polymerase (Kapa Biosystems, https://www.kapabiosystems.com/region-selector/), 50–200 ng of DNA template, and PCR primers (Table 2) and operated under various cycling conditions. We confirmed FeLV status in our laboratory by using FeLV-PCR and antigen ELISA tests and established protocols (28,29).
We extracted PCR products after electrophoresis from a 0.7% agarose gel, purified them by using a MEGAquick-spin Total Fragment DNA Purification Kit (iNtRON Biotechnology, http://jhscience.com/index.php?manufacturers_id=1), and cloned them into a pJET 1.2 blunt vector by using the CloneJET PCR Cloning Kit (Thermo Fisher Scientific, https://www.thermofisher.com/us/en/home.html). We transformed plasmids into XL1-Blue E. coli competent cells (Agilent, https://www.agilent.com). Positive clones were prepared by using DNA-Spin Plasmid Purification Kit (iNtRON Biotechnology) and plasmids. Sanger sequencing used primer walking (Quintarabio, https://www.quintarabio.com). Chromatograms were verified visually to ensure that bases were scored correctly. Full genomes were assembled by using de novo assembly in Geneious version 7.0.6 (https://www.geneious.com).
Because of sample autolysis, full FeLV genomes from 4 panthers (2 contemporary, UCFP228 and UCFP269, and 2 historic, FP115 and FP132) and 1 domestic cat from Florida (x1608) were not recoverable. Partial genome sequencing was performed by using 3 fragments in the gag and env genes (Table 1). We developed forward and reverse primers to sequence a 115-bp long terminal repeat (LTR)–gag fragment, a 98-bp gag fragment, and a 121-bp env fragment (Table 2).
FeLV-B Screening Assay
Phylogenetic Analysis
We analyzed full-genome and partial env (1–1,294 nt) sequence datasets separately. We compared Florida panther FeLV (FeLV-Pco) and domestic cat FeLV (FeLV-Fca) sequence identity by using the SDTv1.2 nt pairwise comparison tool (31). A partial env tree was drawn to include as many GenBank FeLV sequences as possible. We aligned the 3 datasets (full genome, concatenated partial genome [3 small segments within the LTR-gag, gag, and env regions from FP115, UCFP228, and UCFP269], and x1608 along with those comparable regions from the FeLV full genomes available, and env) by using MUSCLE in MEGA version 5.3 (https://www.megasoftware.net) and manually checked open-reading frames (32,33).
To investigate phylogeny, we constructed a midpoint-rooted maximum-likelihood nucleotide tree for the full genome and concatenated partial dataset by using 1,000 bootstrap replicates. We determined best-fit substitution models by using jModelTest (34) in MEGA version 5.3 and phylogenetic trees constructed in PhyML implemented in SeaView4 (35) for the full-genome dataset TN93 + G model (36) and the concatenated partial sequencing nucleotide K2 + G model (37). A neighbor-joining tree constructed for the env dataset by using SeaView4 with a Jukes-Cantor substitution model was rooted with enFeLV and FeLV-B env genetic sequences (38).
Recombination was not removed from env sequences before constructing the tree to clearly demonstrate the phylogenetic relationship of FeLV-B in relation to enFeLV and FeLV-A. For all phylogenetic trees, branches with support <60% were collapsed. Full genomes from UCFP149, UCFP149R1, UCFP231, UCFP275, x1613, and partial sequences (LTR-gag, gag and env) from FP115, FP132 (3,688 to 8,396 nt), UCFP228, UCFP269, and x1608 are available in GenBank (30) (Table 1). Pairwise identity of full genomes were compared for FeLV-Pco, FeLV-Fca, and FeLV-B (GenBank accession nos. JF957361 and JF957363; isolated in the United Kingdom) by using Sequence Demarcation Tool version 1.2 (31).
FeLV Diagnosis and Case Attributes
We determined prevalence and 95% CIs for FeLV diagnosed in Florida Panthers during 3 periods (Table 3). FeLV was first detected in the Florida panther in 2001 (19,39). This outbreak affected panthers residing mainly in protected areas in Florida (Figure 1), including Florida Panther National Wildlife Refuge, Big Cypress Swamp, and Okaloacoochee Slough State Forest (24). During 2001–2004, ≈131 animals were tested for FeLV, and 5 (3.82%) were found to be FeLV antigen positive (19). During 2004–2010, ≈125 animals were negative. The first FeLV case in Florida panthers after the initial outbreak was documented in a road-killed panther in December 2010. During 2014–2016, five additional FeLV-positive free-ranging Florida panthers were identified west of the historic outbreak in more populated areas (Figure 1, panel B). One of these panthers (UCFP231) might have died from infection with FeLV. Cause of death for the other 5 panthers was collision with a vehicle. Contemporary cases identified during 2010–2016 (6 of 184) primarily represented animals hit by vehicles in human populated areas (Figure 1). Prevalence (3.26%) was similar to prevalence during 2001–2004 (Table 3). Lymphadenopathy was documented in 2 of 6 animals (UCFP149, UCFP275). Animal UCFP149 had a linear stomach ulcer. The other animals were too autolyzed to identify gross abnormalities. Confirmatory FeLV PCR and antigen ELISAs reestablished positive and negative diagnoses for the contemporary outbreak.
Identification of Recombinant Subgroup FeLV-B
A clone sequenced from sample UCFP241 was identified as the recombinant subgroup FeLV-B. An env alignment of FeLV-Pco, FeLV-Fca, and enFeLV (Appendix Figure 1) confirmed an infection of FeLV-B resulting from a previously reported recombination event between FeLV-A and enFeLV. Multiple clones were sequenced to confirm presence of FeLV-B. All other Florida panther samples were screened for FeLV-B by PCR (28) but were negative.
Pairwise Comparison and Phylogenetic Analysis
FeLV-Pco showed ≈75% identity at the nucleotide level with published FeLV-Fca and ≈94%–98% identity at the nucleotide level to with FeLV isolated from domestic cats in Florida (x1613, x2653, and x2655). The Florida panther FeLV-B full genome sequenced from x2272 showed ≈75% identity with sequenced FeLV-B and 95%–97% identity with FeLV-Pco subgroup A viruses (AppendixFigure 2).
Full-Genome Phylogeny
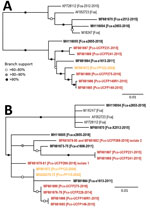
Figure 3. Maximum-likelihood phylogenetic trees showing 2 distinct FeLV-Pco clades in Florida panthers, Florida, USA. A) Full-genome phylogeny indicates Florida FeLV-A sequences are monophyletic. Historic and contemporary FeLV outbreak sequences reside in 1...
The maximum-likelihood tree of full FeLV-Pco and FeLV-Fca genomes documents 2 monophyletic linages: a lineage composed of FeLV isolates Florida (FeLV-Fca and FeLV-Pco) and a lineage composed of published FeLV-Fca isolates from other geographically distinct locations in the United Kingdom and the United States, including 1 FeLV-Fca isolate from Florida. FeLV-Fca isolate (x2655) from Florida groups basal to the 2 FeLV-Pco clades but within the larger Florida FeLV linage. FeLV-Fca isolate x1613 is basal to the clade that includes all historic and related contemporary isolates. FeLV-Fca isolate x2512 was recovered as part of this study originating from Maryland and groups with FeLV-Fca isolate x2653 as part of a relatively homogenous United States/United Kingdom clade (Figure 3, panel A).
FeLV-Pco isolates fall into >2 clades. Two isolates from the contemporary outbreak are monophyletic (UCFP231 and UCFP241) and are referred to contemporary FeLV-Pco. The other FeLV-Pco clade contains 2 FeLV-Pco isolates from the contemporary outbreak (UCFP149 and UCFP275) and is most closely related to the historic FeLV-Pco isolate FP122; this group is referred to here as historic FeLV-Pco.
Partial Genotype Phylogeny
Partial genotyping FeLV-Pco sequences documented 10 single-nucleotide polymorphisms (SNPs) and a 9-nt insertion in the untranslated LTR-gag region, 3 SNPs in the gag region, and 8 SNPs in the env region. Phylogenetic analysis of the short concatenated sequence supported similar relationships established by full-genome nucleotide trees (Figure 3, panel B). An additional historic FeLV-Pco (FP115) is most related to other historic FeLV-Pco isolates. FeLV-Pco from a contemporary outbreak sample (UCFP228) also falls in the historic FeLV-Pco clade. Five clones sequenced from the env portion of FeLV-Pco UCFP269 showed 2 genotypes. Concatenated sequences from UCFP269 showed a paraphyletic relationship (Figure 3, panel B). Both isolates clustered outside the historic and contemporary FeLV-Pco strain clades (Figure 3, panel B). FeLV-Fca (x1608) groups within the major Florida FeLV clade (Figure 3, panel B).
Phylogeny of env
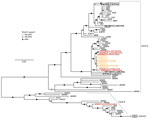
Figure 4. Env phylogenies supporting relationships established in the full-genome tree and document FeLV-B-Pco relationship to other known recombinant viruses in Florida panthers, Florida, USA. The env tree shows FeLV-A, FeLV-B, and enFeLV...
Phylogenetic relationships established by full-genome and concatenated partial sequence trees were supported by the env neighbor-joining tree (Figure 4). The env phylogeny clearly shows differentiation between FeLV-A/FeLV-B/enFeLV subgroups. FeLV-B and enFeLV subgroups cluster together, and FeLV-A remained a monophyletic group. All FeLV-Pco sequences detected during the historic outbreak are monophyletic (24). Two env sequences described in this study are closely related to previous sequences reported from the same animal. FP132, an isolate from a panther sample obtained in 2004, has 2 SNPs; FP122, also obtained in 2004 has 5 SNPs. FeLV-Pco sequences clustered into 2 clades, supporting previously identified relationships. One FeLV-Pco clone (UCFP241B) clusters in the FeLV-B clade.
Two distinct FeLV outbreaks were recorded in the Florida panther population during 2001–2016 (Table 3). Phylogenetic data from both outbreaks documents that FeLV-Pco resulted from initial spillover from domestic cats, presumably during a predatory event (24,39). Although FeLV prevalence in domestic cats is relatively low (≈4%) in Florida (40), it has been reported that domestic cats are ≈5% of Florida panther diet (M. Cunningham et al., unpub. data). The relatively high number of domestic cats consumed, particularly in panther habitat proximal to human development, presents opportunities for FeLV spillover from cats to panthers (40).
Genomic analysis of contemporary FeLV-Pco identified 3 independent isolates (UCFP149, UCFP149R1, and CFP275) that were genetically similar to historic FeLV-Pco (FP122; Figure 3, panel A). Analysis also detected FeLV in a paraphyletic clade that is similar to, but distinct from, historic FeLV-Pco (>97.0% nt identity with FP122 (Appendix Figure 2). This genotype was detected in 2 panthers sampled in 2015 (UCFP231, UCFP241; Figure 3, panel A). A third FeLV strain might be present in contemporary samples and represented by partial FeLV sequences derived from 1 panther (UCFP269; Figure 3, panel B). Three Florida FeLV-Fca isolates (x1613, x2655, and x1608) clustered with Florida panther genotypes. One Florida FeLV-Fca (x2653) isolate was strongly divergent from other Florida FeLV-Fca isolates and resembled previously characterized FeLV-61E and domestic cat FeLV isolates from the United States and the United Kingdom. Movement of domestic cats by owners likely results in mixing of FeLV strains beyond geographic sites.
Full-genome and concatenated partial genome trees demonstrate that domestic cat FeLV strains are situated basal to FeLV-Pco, providing evidence of a domestic cat origin of the panther FeLV infections. Genetic distances between these Florida FeLV-Fca isolates and FeLV-Fca isolates from locations other than Florida indicate a more distant evolutionary relationship between domestic cat strains and Florida FeLV-Fca and FeLV-Pco strains (Figure 3). These findings suggest that minimal species adaptation is required for cross-species transmission between cats and panthers. Additional FeLV full genome samples would enable a Bayesian ancestral reconstruction analyses to further resolve FeLV isolate ancestry.
Full-genome phylogenetic analysis supports the combination and hybrid panther FeLV reemergence hypotheses (Figure 2, panels C, D). Assuming that the Florida panther population was ≈300 animals during 2004–2010, sampling 125 of these animals with no FeLV detected provides a >95% CI that FeLV prevalence was <3%. Test results during both outbreak periods indicated an FeLV prevalence of ≈3%, which indicated that control measures initiated during the historic outbreak were successful in at least controlling, if not eliminating, additional panther FeLV infections for several years. Contemporary FeLV in Florida panthers was identified near human population centers where exposure to feral domestic cats would be more likely to occur (41). Although it is feasible that each contemporary case represented an individual exposure to a different domestic cat, panther-to-panther transmission cannot be excluded, particularly for cases that occurred around the same time and showed similar genotypes (i.e., UCFP231 and UCFP241 sampled in 2015; UCFP228 sampled in 2014; and UCFP275 sampled in 2016).
In addition to the common horizontally transmissible FeLV subgroup (FeLV-A), we recovered and sequenced an oncogenic FeLV subgroup (FeLV-B) from tissues from a contemporary Florida panther (UCFP241B; Figure 3, panel B; Appendix Figure 1). This subgroup is a recombinant of FeLV-A and enFeLV, an endogenous retrovirus harbored only by members of the genus Felis. Identification of FeLV-B infection in a Florida panther is only possible as a result of horizontal transmission of FeLV from a domestic cat because panthers lack enFeLV to support recombination (22). FeLV-B is common in domestic cats; recombination occurs in ≈33%–68% of cats infected with FeLV-A (42), presumably by independent recombination events that occur de novo after infection of domestic cats with FeLV-A.
FeLV-B horizontal transmission has been described only on 3 previous occasions (43). One study reported that FeLV-B was detected in a jaguar (Panthera onca); however, this analysis was based upon sequence amplified from a 232-bp region of the LTR (12,21). We have clearly documented a full FeLV-B genomic sequence in an endangered non-Felis cat species. This finding is of concern because FeLV-B is oncogenic and associated with increased illness and death in domestic cats (6,44,45). Because non–Felis spp. cats lack enFeLV, they might be more vulnerable to an adapted FeLV-B that is readily horizontally transmitted between animals. Thus, spillover of FeLV-B from domestic cats co-infected with this recombinant strain could represent a greater risk for vulnerable nondomestic cat populations.
Besides individual and population health effects, a potential outcome of FeLV infection in nondomestic felids is germline infection leading to endogenization. Early endogenization results in an infection in which the virus has yet to accumulate mutations rendering the endogenous retrovirus defective; therefore, at this stage, the virus might be passed horizontally to other animals (46). Early endogenization might result in decreased fitness, as exemplified by endogenization of koala endogenous retrovirus (46,47). Infection with this virus has also led to higher incidence of secondary infections, such as chlamydiosis and neoplasias (48). Therefore, FeLV infection of panthers and other non-Felis cat species is a greater concern for long-term population effects.
Our study demonstrated that even with efforts to control FeLV in an intensively managed population, FeLV remains a risk to Florida panthers, particularly for animals inhabiting areas near urban centers. Moss et al. reported that the proportion of diet consisting of domestic animals is increasing for Colorado pumas, which is concurrent with puma co-localization in human habitats (49). This trend is likely present in in Florida.
This report highlights the need for continued surveillance of Florida panthers for exposure to FeLV as a major risk management strategy (40). Annual sampling of a proportion of the Florida panther population that is sufficient to detect an FeLV incidence of 3% with relative certainty and increased vaccination of panthers and domestic cats along sites of potential interaction are recommended measures to protect against future outbreaks.
Dr. Chiu is a veterinarian at Colorado State University, Fort Collins, CO. His research interests include virology, disease ecology, and wildlife conservation medicine.
Acknowledgments
We thank the field biologists and veterinarians at the Florida Fish and Wildlife Conservation Commission and National Park Service for their assistance with obtaining sample collections and Jenn Malmberg for helping create the map in this publication.
This study was supported by National Science Foundation–Ecology of Infectious Diseases (award no. 1413925) and the Office of the Director, National Institutes of Health (award nos. T32OD012201 and F30OD023386).
References
- Stewart MA, Warnock M, Wheeler A, Wilkie N, Mullins JI, Onions DE, et al. Nucleotide sequences of a feline leukemia virus subgroup A envelope gene and long terminal repeat and evidence for the recombinational origin of subgroup B viruses. J Virol. 1986;58:825–34.PubMed
- O’Hara B, Johann SV, Klinger HP, Blair DG, Rubinson H, Dunn KJ, et al. Characterization of a human gene conferring sensitivity to infection by gibbon ape leukemia virus. Cell Growth Differ. 1990;1:119–27.PubMed
- Takeuchi Y, Vile RG, Simpson G, O’Hara B, Collins MK, Weiss RA. Feline leukemia virus subgroup B uses the same cell surface receptor as gibbon ape leukemia virus. J Virol. 1992;66:1219–22.PubMed
- Hoover EA, Mullins JI. Feline leukemia virus infection and diseases. J Am Vet Med Assoc. 1991;199:1287–97.PubMed
- Fan H, Gulley ML. DNA extraction from fresh or frozen tissues. In: Killeen AA, editor. Molecular pathology protocols. Totowa (NJ): Humana Press Inc.; 2001. p. 5–10.
- Posada D. Selection of models of DNA evolution with jModelTest. Totowa (NJ): Humana Press Inc; 2009.
- Tavaré S. Some probabilistic and statistical problems in the analysis of DNA sequences. Lect Math Life Sci. 1986;17:57–86.
- Jukes TH, Cantor CR. Evolution of protein molecules. New York: Academic Press; 1969.
- Aguilar GD, Farnworth MJ. Distribution characteristics of unmanaged cat colonies over a 20 year period in Auckland, New Zealand. Appl Geogr. 2013;37:160–7. DOI
Figures
Tables
Suggested citation for this article: Chiu ES, Kraberger S, Cunningham M, Cusack L, Roelke M, VandeWoude S. Multiple introductions of domestic feline leukemia virus in endangered Florida panthers. Emerg Infect Dis. 2019 Jan [date cited]. https://doi.org/10.3201/eid2501.181347
Original Publication Date: 11/15/2018
1Preliminary results from this study were presented at the Ecology and Evolution of Infectious Diseases Conference, June 24–27, 2017, Santa Barbara, California, USA.
No hay comentarios:
Publicar un comentario