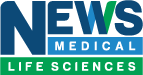
Producing Super-Swelled Lyotropic Monocrystals for Drug Development
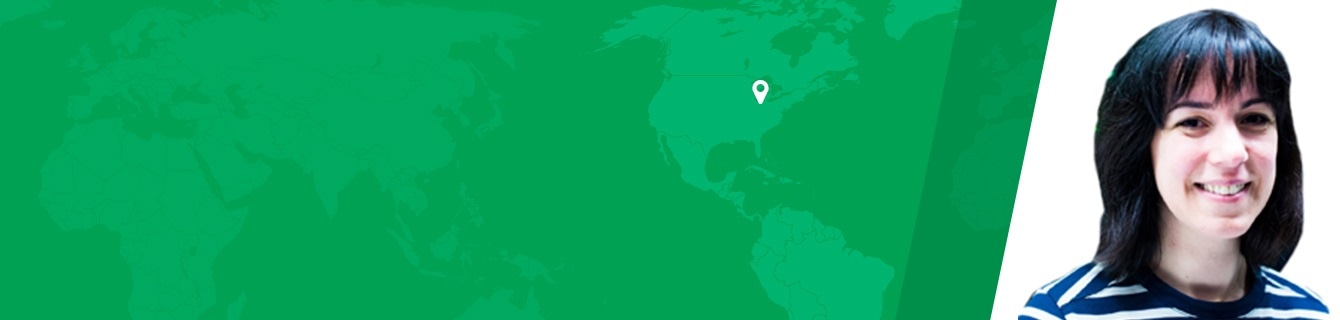
What are lyotropic liquid crystals? How are they used in drug development?
Liquid crystals are state of matter that have properties of liquids and crystals. There are several types of liquid crystal materials. There are some that change their properties as a response to temperature, called thermotropic liquid crystals, and others that change in response to a solvent. These are called lyotropic liquid crystals and in most cases the solvent is water.
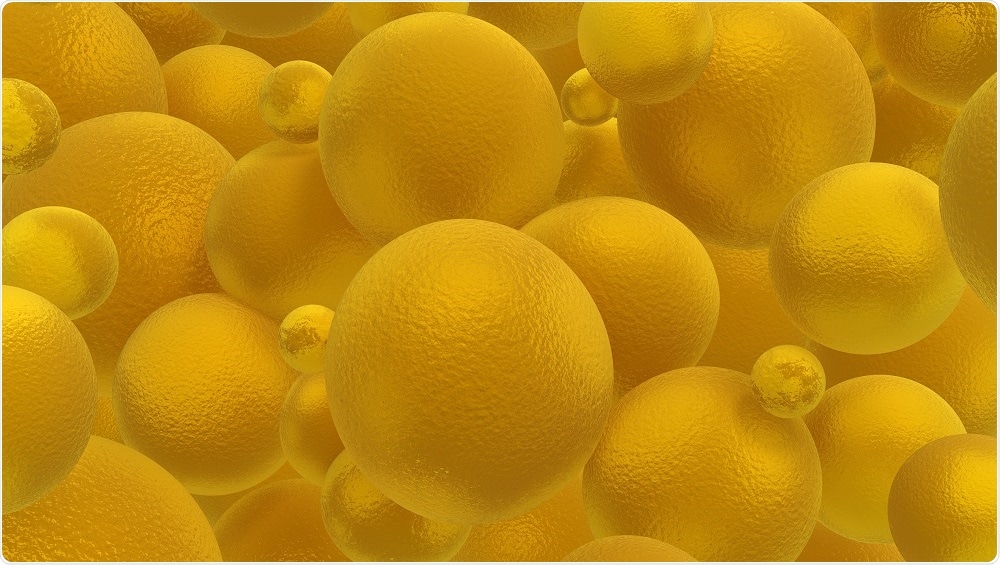
Lyotropic liquid crystals are used in drug development because they are liquids, however, they do have some crystalline properties. There useful in drug development as they can be organized into structures that are ideal to encapsulate drugs and other bioactive molecules.
Lyotropic liquid crystals are used because they remain stable in water, which makes up a large percentage of the human body. Aqueous structures are essential for drug delivery in vivo, which is why lyotropic liquid crystals are so useful.
What methodologies have been used to produce lyotropic liquid crystals for drug development in the past?
Liposomes are the most used lyotropic liquid crystal for drug development. They are characterized by a membrane sphere with water and the target drug inside. The way drugs are developed using this method is complex.
You start with a lyotropic liquid crystal, which is a bilayer, or lamellar phase, that looks very much like sheets of a cell membrane. Then, you apply a technique to make little particles come out of the crystal. Following this, you add water and agitate the membrane. This causes the crystal to close-up into spheres with water on the inside; where the drug will go.
However, there are alternative methods. One example is bicontinuous cubic phases, which are three-dimensional objects that have lots of pores. The discovery of bicontinuous cubic phases got scientists thinking, "Well instead of just making liposomes, we can make other types of particles with other types of structures, and that might be beneficial”.
Due to their highly porous nature, these structures can, in theory, hold a larger amount of cargo than liposomes, making them superior structures for drug delivery. But to this day, liposomes are still the leading lyotropic liquid crystal used in drug delivery. This is because bicontinuous cubic phases are difficult to process and their pores are too small.
What are the limitations of the current methods used to produce lyotropic liquid crystals? Why is it important that new methods are developed?
Liposomes are great and very useful, but there are certain drugs that can crystallize inside them. If you have a method that uses a perforated membrane like bicontinuous cubic phases, larger, more complex drugs can be delivered, as the structure has an increased surface to volume ratio.
Another limitation of liposomes is that the drugs need to be soluble. This is a problem for hydrophobic drugs that won’t go into the water domain. They will want to go into the hydrophobic core of the membrane.
In liposomes you only have one membrane that encloses the drug. If you have other phases, like bicontinuous cubic phases, where the particle is made up of perforated membranes that allow the water to enter pores, you have a particle that is a continuum of water and fat everywhere.
If a liposome is, say, 200 nanometers, then those 200 nanometers are mostly made up of water. With bicontinuous cubic phases, you have tons of membranes that are continuous in both polar and hydrophobic domains. This means you can encapsulate drugs that are both water soluble and not water insoluble.
Then there are other advantages. For example, bicontinuous cubic phases are known to have a preferential interaction with cell membranes, allowing easier delivery of drugs into cells of the body.
Please outline your recent research involving lyotropic liquid crystals.
Traditionally, lyotropic liquid crystals had pores that are really small, only about three nanometers. So, there was a limit of what kind of drugs or proteins you could encapsulate within them. Our recent findings describe a new processing technique that allowed us to expand those pores to five times larger than previous methods, so they are now around 15 nanometers in size.
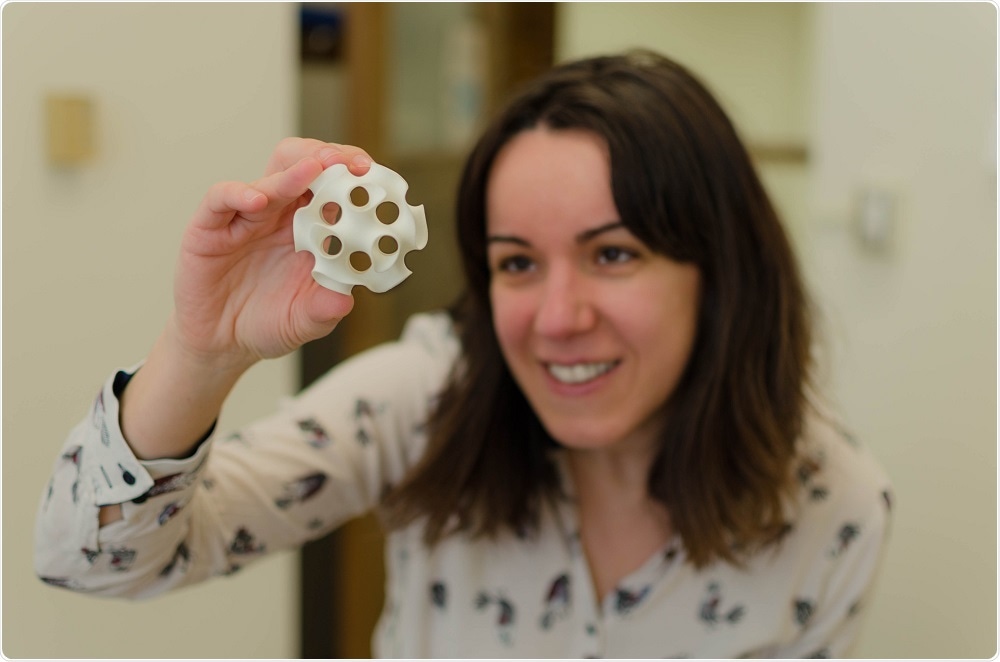
Why are super-swelled lyotropic monocrystals such an advancement?
The first advancement was that we could make these materials with larger pores than ever before. The second was that the materials that we had generated were extremely stable for months. This is because, instead of existing as a collection of membranes, the liquid crystals had formed a monocrystal with extremely large pores.
Typically, if you do anything with membranes, or soft materials in general, you have these small unit cells or these small crystals or micro crystallites that appear. They are the size of a micron and are randomly oriented in all directions in solution.
We've found that if you let these highly porous membranes rest for a few months, they develop into a single crystal that is more than one cubic millimeter. It's no longer micrometer size, its macro size; you can see it with the human eye.
What's surprising to us is that, the crystal is still able to hold a lot of water. It's 90% water, and is still soft but is also a crystalline shape like a diamond. That was very surprising to us. Not only that it is super swollen, but that it was also incredibly well-organized.
Whilst this might not be useful for drug development, it may be useful for protein crystallization. This has actually been used in a recent paper for Nature Communications. The researchers used our method to encapsulate the Gloeobacter ligand-gated ion channel protein (GLIC) when they crystallized it.
How did you find out that fast chloroform removal was crucial for producing lyotropic monocrystals?
If you want to develop a material using a mixture of liposomes or any form of lipid molecules, they first need to be solubilized in an organic solvent. The most commonly used solvent is chloroform, which is removed slowly and completely, creating the lyotropic liquid crystal. The addition of water turns the crystal into a liposome or bicontinuous cubic phase.
My student was working with some of these lipid mixtures, and was in a rush to move to the next step. This meant he removed the chloroform really fast. He then added the water and saw these super swollen phases develop.
He told me he’d messed up but when we looked, the solvent had been completely removed, so it was only the speed that differed, as it is usually done very slowly. He then started working on this method, comparing the size of the phases that develop from a slow, medium and fast speed of chloroform extraction.
He found that when you add water then the size of the pores that develop are a function of the speed at which the solvent is removed. If you do this slowly you get the regular size, and if you do fast you get these super swollen phases.
We assumed it was a kinetic effect that wouldn’t be stable. But, it was stable, not thermodynamically, but it was stable. We let it sit for six months and found that not only was it still stable, it was actually more organized.
In fact, liposomes are not thermodynamically stable either. They are formed via kinetic traps that are stable when you need to apply them but not thermodynamically, therefore this is not a limitation of our discovery. It's been a year since we first discovered this method and our sample is still in the lab today.
What applications are there for super-swelled lyotropic monocrystals? What impact will the new methodology have on research?
I think the major applications of our research will be in protein crystallization. This is important because this is at the forefront of research, with the development of useful technologies such as Cryo-EM and imaging techniques to determine protein structure.
The leading technology to determine protein structure is crystallography. The limitations of current techniques are that most proteins are difficult to crystallize. Before our discovery, the number of proteins that you could crystallize was very limited. This was because the pores were so small that are only a handful of proteins could be crystallized.
In the future, do you think super-swelled lyotropic monocrystals will be routinely used in research and drug development?
Yes, I hope so. The good thing about the method is that it is a relatively easy processing technique, meaning it is accessible to many scientists. It is also a reliable technique as the materials themselves are highly flexible. For example, let’s say you want to use a cocktail of three different lipids that target a specific cell type, with this method, you could use any lipid as long as you removed the solvent fast.
I think that it will have a big impact. However, the widespread use of new techniques take time. People are very used to working with liposomes and they are more familiar than other more complicated structures.
Where can readers find more information?
- Dr. Cecilia Leal’s profile for The University of Illinois
- The Leal Research group
- Zabara, A., Chong, J., Martiel, I., Stark, L., Cromer, B., Speziale, C., Drummond, C. and Mezzenga, R. (2018). Design of ultra-swollen lipidic mesophases for the crystallization of membrane proteins with large extracellular domains. Nature Communications.
About Dr. Cecília Leal
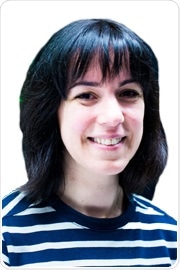
After working for a year in the Norwegian Radium Hospital, she joined Professor Safinya’s Lab at the University of California, Santa Barbara, as a postdoctoral fellow.
Her research interests focus on the characterization and functionalization of lipid materials for cellular delivery. She is the recipient of the National Institutes of Health New Innovator award (2016), the National Science Foundation CAREER award (2016), and the University of Illinois Dean’s Award of Excellence in Research (2018).
No hay comentarios:
Publicar un comentario