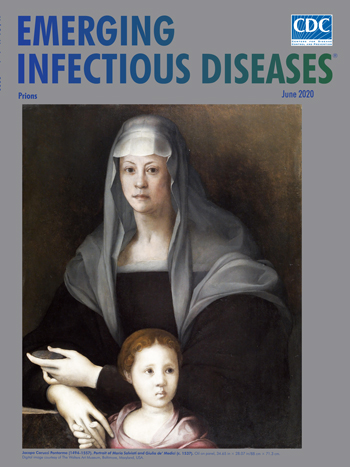
Volume 26, Number 6—June 2020
Research
Zoonotic Vectorborne Pathogens and Ectoparasites of Dogs and Cats in Eastern and Southeast Asia
On This Page
Article Metrics
Vito Colella
, Viet L. Nguyen, Do Y. Tan, Na Lu, Fang Fang, Yin Zhijuan, Jiangwei Wang, Xin Liu, Xinghui Chen, Junyan Dong, Wisnu Nurcahyo, Upik K. Hadi, Virginia Venturina, Kenneth B.Y. Tong, Yi-Lun Tsai, Piyanan Taweethavonsawat, Saruda Tiwananthagorn, Thong Q. Le, Khanh L. Bui, Malaika Watanabe, Puteri A.M.A. Rani, Giada Annoscia, Frédéric Beugnet, Domenico Otranto, and Lénaïg Halos

Abstract
To provide data that can be used to inform treatment and prevention strategies for zoonotic pathogens in animal and human populations, we assessed the occurrence of zoonotic pathogens and their vectors on 2,381 client-owned dogs and cats living in metropolitan areas of 8 countries in eastern and Southeast Asia during 2017–2018. Overall exposure to ectoparasites was 42.4% in dogs and 31.3% in cats. Our data cover a wide geographic distribution of several pathogens, including Leishmania infantum and zoonotic species of filariae, and of animals infested with arthropods known to be vectors of zoonotic pathogens. Because dogs and cats share a common environment with humans, they are likely to be key reservoirs of pathogens that infect persons in the same environment. These results will help epidemiologists and policy makers provide tailored recommendations for future surveillance and prevention strategies.
Asia is the largest continent in the world, known for its thriving biocultural diversity. Today, countries in Asia are experiencing a rapid social, demographic, and economic transformation, thereby placing this region as an ever-growing economic powerhouse in the years to come. Sustained economic growth in Asia has resulted in increased demand for products and services and substantial urbanization (1). These factors have triggered a series of human-mediated environmental alterations, such as deforestation and encroachment of humans into natural ecosystems, that now link previously isolated ecologic niches and give pathogens new opportunities to thrive (2). During the past century, Asia has been in the limelight for emergence and pathogenicity of a large number of infectious diseases that have taken a substantial toll on the health of millions of persons (1). Striking examples include the emergence of severe acute respiratory syndrome, infections with the highly pathogenic avian influenza A(H5N1) virus, and coronavirus disease (COVID-19). Recently, human modification of natural habitats resulted in the emergence of a tick vector of Kyasanur Forest disease virus, a zoonotic vectorborne flavivirus that causes severe hemorrhagic fever with a fatality rate of 3%–10% (3).
Also implicated in the changing epidemiology of pathogens of public health concern in eastern and Southeast Asia are dogs and cats (4–6). In remote areas of eastern and Southeast Asia, three quarters of dogs are classified as stray or community dogs (7). Increases in living standards have led to a dramatic surge in the number of pet dogs and cats living in metropolitan settings (8,9). In China, the population of pet dogs is estimated to grow by 5 million per year. Along with this increase in companion animal ownership, the risk of acquiring parasitic zoonoses from companion dogs and cats represents an ongoing, yet neglected, threat (10,11).
Implementation of effective measures to control zoonotic diseases must rely on the elucidation of pathogens and reservoir hosts in a given area. For most countries in Asia, limited knowledge about the agents parasitizing dogs and cats, including those transmissible to humans, hinders the establishment of proper strategies for treatment and prevention of zoonotic pathogens in animal and human populations. Although previous investigations have explored the occurrence of zoonotic diseases in animals living in remote areas (4–7), our year-long multicenter study explored the occurrence of vectorborne pathogens and ectoparasites in pet dogs and cats from metropolitan areas in eastern and Southeast Asia.
Our study involved academic institutions and private facilities of eastern Asia (China and Taiwan) and Southeast Asia (Indonesia, Malaysia, the Philippines, Singapore, Thailand, and Vietnam). To provide capacity building and compliance with the study procedures, trainings were performed at local institutions as needed. The protocol of this study was approved by the Ethics Committee of the Department of Veterinary Medicine, University of Bari (protocol no. 13/17). At partner institutions, animal owners read, approved, and signed an owner informed consent, which contained information about study procedures.
During 2017–2018, local investigators sampled 10 client-owned dogs and 10 client-owned cats each month for 12 months in each country, except China, where 40 dogs and 40 cats each month were sampled. Inclusion criteria were a history of regular outdoor access and having not received recent antiparasitic treatments. Data on the animals’ location, age, breed, and sex were recorded.
Veterinary Examination
Veterinarians performed a complete examination of the animals, reporting abnormalities in rectal temperature, overall physical condition, demeanor, nasal discharge, skin/haircoat, eyes, superficial lymph nodes, respiratory system (breathing), cardiovascular system (mucous membranes), and fecal consistency. The examinations included checking for the presence of ectoparasites (ticks, fleas, lice, and mites) by examining the whole-body surface for >5 minutes. The veterinarians inspected both eyes, including a thorough examination under the third eyelid to detect adult Thelazia callipaeda eyeworms. They also performed testing for lesions evocative of sarcoptic mange or demodicosis (deep skin scraping), cheyletiellosis (tape test), or otoacariosis (earwax examination).
Sampled parasites were stored in vials containing 70% ethanol and sent for morphologic and molecular identification at the University of Bari (Bari, Italy), where we examined adult and nymph ticks under a stereomicroscope. We clarified tick larvae, fleas, lice, and fur mites in 10% potassium hydroxide overnight, mounted in Hoyer’s medium and observed under an optical microscope (12). We used morphologic keys to identify all ectoparasites to the species level (13–20). For mite identification, we minced crusty skin lesions by using disposable surgical blades, added drops of saline solution on a glass slide, observed the slides under an optical microscope, and identified the mites according to morphologic appearance (18,21). We mounted anterior and posterior extremities of adult T. callipaeda eyeworms in lactophenol and identified them (22).
Molecular Identification of Ectoparasites
To confirm morphologic identifications of ectoparasite species, we subjected a representative subpopulation (≈20%) of the ectoparasites to DNA extraction and amplification of target genes (Appendix Table). For ticks, fleas and lice, we isolated genomic DNA (gDNA) by using the DNeasy Blood and Tissue Kit (QIAGEN, ) according to the manufacturer’s instructions. We isolated gDNA from a small portion of the idiosoma of ticks (23) and from the anterior dorsal part of the abdomen of fleas (24). We selected individual lice and mites under an optical microscope and extracted gDNA by using a QIAamp DNA Micro Kit (QIAGEN).
Blood Collection and Processing
From each study animal, we collected ≈2 mL of blood in a tube with anticoagulant and processed it as follows. For dogs, we used an aliquot of the blood sample for the ELISA-based technology SNAP 4Dx Plus test (IDEXX Laboratories, Inc., >6 h) at room temperature for blood to dry, and put them in a zip-locked plastic bag.
) to detect Dirofilaria immitis antigen and antibodies against Anaplasma phagocytophilum/A. platys, Borrelia burgdorferi sensu lato, and Ehrlichia canis/E. ewingii as described and the SNAP Leishmania test (IDEXX Laboratories, Inc.) to detect antibodies against Leishmania infantum/L. donovani as described. For cats, we used an aliquot of blood to detect antigens of feline leukemia virus (FeLV) and D. immitis and antibodies against feline immunodeficiency virus (FIV). We used the SNAP Combo FIV/FeLV and SNAP Heartworm RT Test (IDEXX Laboratories, Inc.). For dogs and cats, we blotted 2 spots of blood (125 μL each, total 250 μL/animal) onto Whatman FTA cards (Sigma-Aldrich Corp., ), stored the cards overnight (DNA Extraction, Amplification, Purification, and Sequencing
From each Whatman FTA card, we punched out 5 disks of 3.0-mm each (Uni-Core 150 punch; GE Healthcare, Appendix Table). We detected Leishmania protozoa by quantitative PCR (qPCR) and further tested only samples scoring positive in duplicates by qPCR by cPCR on the internal transcribed spacer 2 region and kinetoplast DNA for species identification (Appendix Table).
) and placed them in each well of a 96-well plate (QIAcube HT kit Plasticware; QIAGEN) and included a negative control (Whatman FTA card blotted with dog blood naive to the pathogens in this study) for each plate. Subsequently, we added a 200-μL solution (180 μL of buffer ATL and 20 μL of proteinase K) to each well and subjected samples to prelysate overnight incubation at 56°C in a 711 CT incubator (Asal s.r.l., ). We extracted DNA by using a QIAcube HT and the QIAamp 96 DNA QIAcube HT kit (QIAGEN) according to manufacturer instructions. We tested all gDNA isolated from dried blood samples by conventional PCR (cPCR) (For all PCRs, we included positive controls (DNA of pathogen-positive blood samples) and negative controls (DNA of pathogen-negative blood samples). We visualized PCR amplicons from nematodes and apicomplexan protozoa by capillary electrophoresis by using a QIAxcel DNA screening gel cartridge on a QIAxcel system (QIAGEN for each) and used a QX DNA Size Marker (QIAGEN) to size PCR products. We injected a QX Alignment Marker (QIAGEN), which consisted of 15-bp and 3,000-bp fragments, onto the cartridge with each sample. We then determined the PCR product sizes by using QIAxcel Screen Gel 1.4.0 software (QIAGEN). We subjected cPCR products from Leishmania spp. protozoa, Thelazia spp. eyeworms, ticks, fleas, lice, and mites to electrophoresis in a 2% agarose gel stained with Gel Red (VWR International PBI,
) and visualized them on a Kodak Gel Logic 100 gel documentation system ( ).We purified all cPCR amplicons obtained and sequenced them in both directions in an automated sequencer ABI-PRISM 377 (ThermoFisher Scientific,
). We edited and aligned the sequences by using Geneious Prime software ( ) and compared them with each other and with those available in the GenBank database by using BLAST ( ).Statistical Analyses
We calculated frequency values as the proportion of positive animals to the total number of examined animals and the relative frequency of occurrence of each species of parasite as the proportion of animals infested by a given parasite species/group within the total number of positive results within a given parasite species/group. We calculated 95% CIs by using the Wilson score interval.
We categorized animals into 3 age groups (<1, >1 to <5, and >5 years). We used the χ2 test to investigate associations between parasitic infection/exposure or infestation by ectoparasites and age group or clinical observations. We analyzed the Cohen κ coefficient and dependent and independent variables by using GraphPad Prism 8 ( ). We considered p<0.01 to indicate significance.
Our study sample consisted of 2,381 animals (1,229 dogs and 1,152 cats). Samples were collected from animals living in 23 main cities (and neighboring localities) in 8 countries in Asia, specifically China (Beijing, Nanjing, Shanghai, and Guangxi Province), Taiwan (Taipei, Taoyuan, Changhua, Pingtung, and Hualien), Indonesia (Jakarta, Bogor, and Yogyakarta), Malaysia (Klang Valley region and Kota Bharu), the Philippines (Cabanatuan, San Jose, and Munoz), Singapore, Thailand (Bangkok and Chiang Mai), and Vietnam (Hanoi and Ho Chi Minh City) (Figure 1).
The dog population was composed of 565 (46.0%) females, 660 (53.7%) males, and 4 (0.3%) with unreported data; the cat population was composed of 543 (47.1%) females, 606 (52.6%) males, and 3 (0.3%) with unreported data. Ages of dogs ranged from 2 months to 20 years (mean 5.1 years, median 4.0 years), and ages of cats ranged from 2 months to 20 years (mean 2.7 years, median 2.0 years) (Table 1).
Overall, 42.4% (95% CI 39.7%–45.2%) of dogs and 31.3% (95% CI 28.4%–33.7%) of cats had >1 ectoparasite detected, exposure to vectorborne parasites, or both (Tables 2,3,4,5,6; Figures 2,3,4,5,6). In particular, 33.5% (95% CI 30.9%–36.2%) of dogs and 31.3% (95% CI 28.4%–34.0%) of cats were infested with >1 ectoparasite, and 22.8% (95% CI 20.5%–25.2%) of dogs and 0.5% (95% CI 0.2%–1.1%) of cats were detected with or exposed to >1 vectorborne parasite. T. callipaeda eyeworms were detected in 1.7% (95% CI 0.8%–3.3%) of dogs from China, specifically in 6.7% (95% CI 3.4–12.7) of dogs and 0.6% (95% CI 0.2%–1.8%) of cats from Beijing.
We detected co-infections with Hepatozoon canis and D. immitis heartworms in 4 dogs (2 each from Thailand and the Philippines). No tick infestations were found on dogs infected with B. gibsoni, but Rhipicephalus sanguineus ticks were detected on 50% of animals with H. canis infection. H. canis infection was correlated with infestation by R. sanguineus ticks (p = 0.0125).
A total of 4 dogs (0.3%, 95% CI 0.1%–0.8%) were positive for antibodies against Leishmania infantum; 2 of these dogs were from China (0.4%, 95% CI 0.1%–1.5%) and 1 each from Vietnam (0.8%, 95% CI 0.1%–4.6%) and the Philippines (0.9%, 95% CI 0.2%–5.0%). In addition, the 2 seropositive dogs from China were positive for L. infantum by qPCR and cPCR Sanger sequencing (Table 6).
A total of 2 dogs (0.2%, 95% CI 0–0.6) were positive for antibodies against Borrelia burgdorferi sensu lato. One was in the Philippines (0.9%, 95% CI 0.2%–4.9%), and 1 was in Indonesia (1.1%, 95% CI 0.2%–5.7%).
Overall, 5.2% (95% CI 4.1%–6.7%) of dogs were infected with filarial parasites according to antigen testing (3.5%, 95% CI 2.6%–4.6%), cPCR (2.7%, 95% CI 1.9%–3.7%), or both. cPCR-coupled sequencing identified Brugia spp. in 0.4% of dogs (95% CI 0.2%–0.9%) and in 15.2% (95% CI 6.6%–30.9%) of the samples positive for filariae by cPCR. Specifically, B. pahangi was found in dogs in Thailand (1.7%, 95% CI 0.5%–5.9%) and Malaysia (2.2%, 95% CI 0.4–11.6), and B. malayi was found in dogs in Vietnam (0.8%, 95% CI 0.1–4.6) and Thailand (0.8%, 95% CI 0.1%–4.6%). Using the kappa statistic, we found slight to fair agreement between antigen testing and cPCR (κ = 0.271, 95% CI 0.079–0.463) for the diagnosis of D. immitis in dogs. One cat (1.3%, 95% CI 0.2%–6.9%) from Indonesia was positive for D. immitis antigen.
H. canis infection was diagnosed for 1 cat from the Philippines (0.9%, 95% CI 0.2–5.1) and B. gibsoni for 3 cats in China (0.6%, 95% CI 0.2%–1.8%) and 1 cat in Singapore (0.8%, 95% CI 0.1–4.3). FIV antibodies were detected in 5.2% (95% CI 4.0%–6.6%) of cats and FeLV antigens in 2.9% (95% CI 2.1%–4.0%).
We compiled statistically significant associations for the detection of/exposure to >1 ectoparasite or vectorborne pathogen, to ectoparasites or vectorborne parasites only, and to filarial parasites in dogs in different age classes (Appendix Figure 1). The finding of clinical signs (e.g., respiratory, lymph nodes, ocular, and skin abnormalities and increased body temperature) was statistically associated with the overall detection of/exposure to >1 parasite (Appendix Figure 2) and with ectoparasite infestation or detection of/exposure to vectorborne parasites in dogs (Appendix Figure 3). For cats, we found no association between age group and detection of parasites, whereas clinical signs (i.e., enlarged lymph nodes and skin abnormalities) were statistically associated with detection of ectoparasitic infestation (Appendix Figure 4). We found no statistical association between seropositivity for FIV antibodies and FeLV antigens and detection of ectoparasites, vectorborne pathogens, or both.
The detection of zoonotic pathogens in client-owned dogs and cats living in metropolitan areas indicates that these animals serve as hosts for several parasitic agents in Asia. We provide data for an extended geographic distribution of zoonotic pathogens (e.g., L. infantum protozoa and zoonotic species of filariae) and of arthropods infesting animals (e.g., ticks of the Haemaphysalis and Rhipicephalus genera) where prior data unavailability made treatment and disease control strategies unachievable.
Nearly half of the dogs and one third of the cats in this study were infested with >1 ectoparasite or exposed to vectorborne pathogens; prevalence peaked in countries with a humid tropical climate (e.g., the Philippines, where 67% of dogs were infested with ticks, and Malaysia, where 89% of cats were infested with fleas). Such findings raise concern that vectorborne pathogens are responsible for several zoonotic diseases in Southeast Asia (25). The most prevalent tick on dogs and cats in this study was R. sanguineus. The taxonomic status of this tick group is a matter of debate with regard to R. sanguineus sensu lato including 2 lineages, so-called temperate and tropical (26–28). The tropical lineage of the R. sanguineus s.l. tick is prevalent in most countries in Asia and has been deemed accountable for the transmission of pathogens causing babesiosis, ehrlichiosis, and several rickettsial diseases in Asia (25,29,30). Despite the high proportion of tick-infested animals, the paucity of data on the ecology of R. sanguineus s.l. ticks in Asia makes their role as a vector difficult to ascertain.
Unexpectedly, we found tick species not classically associated with companion animals but with the potential to transmit zoonotic disease–causing pathogens in dogs. For example, Haemaphysalis hystricis ticks have been implicated as vectors of a novel Borrelia species closely related to the relapsing fever group (31), and Haemaphysalis wellingtoni ticks are vectors of Kyasanur Forest disease virus, which causes fatal epidemics among monkeys and leads to hospitalization of ≈500 persons/year in India (3). Moreover, dogs seropositive to B. burgdorferi s.l. in this study were from Indonesia and the Philippines. This finding is unexpected, considering that these bacteria have been detected outside the known distribution area of Ixodes tick species, the main vectors of B. burgdorferi s.l., and indicates a need for in-depth epidemiologic surveys of this group of pathogens in Southeast Asia.
These results update the list of pathogens and ectoparasites affecting companion animals in Asia, including ticks with multihost feeding behavior, which has the potential to extend the network of pathogen transmission further into urban areas. The same holds true for pet dogs, suggesting that these animals might have been overlooked as potential pathogen reservoirs in metropolitan settings in this geographic area.
Similarly, the Ctenocephalides orientis flea was identified in one fifth of flea-positive dogs. The host spectrum of this flea is wider, but apparently its geographic distribution is more limited than that of the well-known cat flea Ctenocephalides felis, and it is involved in the transmission of rickettsiae, including Rickettsia sp. genotype RF2125 and Rickettsia sp. TH2014 (32,33). The morphologic ambiguity of the C. orientis flea (probably misidentified as Ctenocephalides canis and previously reported as a subspecies of C. felis) has contributed to a substantial dearth of information on its global distribution and role as a vector. In contrast, the cosmopolitan C. felis flea has colonized different bioclimatic niches, mainly through human-mediated migration (34). As human and animal global transportation increase in Asia, constant vigilance regarding the introduction of C. orientis fleas outside their known range of distribution in developed and developing countries is essential, as supported by the recent report of detection of fleas of this species in Iran (35).
In Singapore, one of the countries with the highest human development index (36) and lowest proportion of animals affected by parasites, Lynxacarus radovskyi, a mite for which little is known regarding its ecology, was detected on 35% of sampled cats. We have provided molecular data and updated morphologic information on this listophorid mite, which is an agent of papular dermatitis in humans (37). Furthermore, availability of appropriate diagnostics for this species or data on the efficacy of ectoparasiticides against it are limited.
Refined diagnostics are essential for assessing the distribution of filarial species in canine populations. For instance, the poor agreement (κ = 0.271) between cPCR and antigen-detection tests for D. immitis advocates for the use of integrated diagnostics to better appreciate the epidemiologic status of this species of filariae. Furthermore, the use of both tests revealed B. pahangi and B. malayi to also (in addition to D. immitis) affect companion animals in the regions investigated. These 3 species of filariae cause clinical manifestations in humans: lymphatic filariosis for B. malayi and B. pahangi (38,39) and pulmonary granulomas for D. immitis (40). In particular, lymphatic filariosis is among the most debilitating neglected tropical diseases; an estimated 70 million persons are infected, among which >50% live in Southeast Asia (41,42), and D. immitis infection of humans poses significant diagnostic challenges (40). Hence, for development and enactment of global elimination programs (41), surveillance of filarial species should be extended to animal populations in filariae-endemic countries (42).
Similarly, Leishmania spp. parasites currently cause ≈500,000 human infections/year in 62 countries (43), although their occurrence in eastern and Southeast Asia is poorly documented. We detected dogs positive for L. infantum by serology, qPCR, and sequencing in China and seropositive dogs in Thailand and Vietnam. In Thailand, the recent emergence of L. martiniquensis and L. siamensis caused immunocompetent and immunocompromised persons to seek medical assistance (44). A range of animals is involved in the zoonotic cycle of these 2 species (44,45), but dogs are the main reservoir for zoonotic leishmaniosis caused by L. infantum (46). The role of Leishmania spp. in human infections and as agents of disease in Southeast Asia requires urgent attention.
Further complicating knowledge of the transmission of zoonotic parasites in these regions of Asia are the large populations of free-roaming animals; the increased number of pet dogs and cats; and the complex social, economic, and ecologic changes currently occurring in Asia, (1,2,4,25,47,48). Integrated strategies that address all of these factors are therefore fundamental for the control of such parasitic agents. We investigated the presence of pathogens and ectoparasites in pet dogs and cats living in metropolitan areas in close proximity to humans. These animals share a common environment with humans, which makes them likely key reservoirs for pathogens with the potential to infect persons living in such areas and settings.
The epidemiologic data presented in this study can be pivotal for building knowledge bases about the occurrence of zoonotic parasites infecting companion dogs and cats in eastern and Southeast Asia. This information could help epidemiologists and policy makers provide tailored recommendations in the blueprint of future surveillance and prevention strategies.
Dr. Colella is a postdoctoral research fellow at the University of Melbourne, Australia. His main research is focused on the development of epidemiologic studies and strategies for interventions against zoonotic pathogens.
Acknowledgment
We are grateful to Isabelle Richtofen, Jianwei Zhang, Evonne Lim, Clair Cheng, Nadine Duperray, and Marielle Servonnet for their expertise and contributions to managing this logistically challenging study.
References
- Coker RJ, Hunter BM, Rudge JW, Liverani M, Hanvoravongchai P. Emerging infectious diseases in southeast Asia: regional challenges to control. Lancet. 2011;377:599–609.
- Chongsuvivatwong V, Phua KH, Yap MT, Pocock NS, Hashim JH, Chhem R, et al. Health and health-care systems in southeast Asia: diversity and transitions. Lancet. 2011;377:429–37.
- Shah SZ, Jabbar B, Ahmed N, Rehman A, Nasir H, Nadeem S, et al. Epidemiology, pathogenesis, and control of a tick-borne disease—Kyasanur Forest disease: current status and future directions. Front Cell Infect Microbiol. 2018;8:149.
- Conlan JV, Sripa B, Attwood S, Newton PN. A review of parasitic zoonoses in a changing Southeast Asia. Vet Parasitol. 2011;182:22–40.
- Chen J, Xu MJ, Zhou DH, Song HQ, Wang CR, Zhu XQ. Canine and feline parasitic zoonoses in China. Parasit Vectors. 2012;5:152.
- Inokuma H. Panorama of vector borne disease of pets in Asia & Japan. In: Beugnet F, editor. Guide to vector borne diseases of pets. Lyon (France): Merial; 2013. p. 94–107.
- Traub RJ, Irwin P, Dantas-Torres F, Tort GP, Labarthe NV, Inpankaew T, et al. Toward the formation of a Companion Animal Parasite Council for the Tropics (CAPCT). Parasit Vectors. 2015;8:271.
- Dong HP, Liu ZW, Chen H, Zhang LX. The situation and treatment of parasitic zoonoses of pets [in Chinese]. Hennan J Ani Sci. Vet Med. 2007;28:8–10.
- Otranto D, Dantas-Torres F, Mihalca AD, Traub RJ, Lappin M, Baneth G. Zoonotic parasites of sheltered and stray dogs in the era of the global economic and political crisis. Trends Parasitol. 2017;33:813–25.
- Paul M, King L, Carlin EP. Zoonoses of people and their pets: a US perspective on significant pet-associated parasitic diseases. Trends Parasitol. 2010;26:153–4.
- Chomel BB, Sun B. Zoonoses in the bedroom. Emerg Infect Dis. 2011;17:167–72.
- Gibb TJ, Oseto CY. Arthropod collection and identification: field and laboratory techniques. 1st ed. Boston: Academic Press; 2006.
- Tanskull P, Inlao I. Keys to the adult ticks of Haemaphysalis Koch, 1844, in Thailand with notes on changes in taxonomy (Acari: Ixodoidea: Ixodidae). J Med Entomol. 1989;26:573–601.
- Hopkins GHE. Siphonaptera. In: Gressitt JL, editor. Insect of Micronesia. Hawaii (HI): Bishop Museum; 1961. p. 91–107.
- Trapido H, Varma MGR, Rajagopalan PK, Singh KRP, Rebello MJ. A guide to the identification of all stages of the Haemaphysalis ticks of South India. Bull Entomol Res. 1964;55:249–70.
- Yamaguti N, Tipton VJ, Keegan HL, Toshioka S. Ticks of Japan, Korea, and the Ryukyu Islands. Brigham Young University Science Bulletin. Biological Series. 1971;15:Article 1.
- Tenorio JM. A new species of Lynxacarus (Acarina: Astigmata: Listrophoridae) from Felis catus in the Hawaiian islands. J Med Entomol. 1974;11:599–604.
- Kettle DS. Medical and Veterinary Entomology. 1st ed. Sydney (Australia): Croom Helm; 1984.
- Lewis RE. Fleas (Siphonaptera). In: Lane RP, Crosskey RW, editors. Medical insects and arachnids. Dordrecht (Netherlands): Springer; 1993. p. 529–575.
- Walker JB, Keirans JE, Horak IG. The genus Rhipicephalus (Acari, Ixodidae): a guide to the brown ticks of the world. Cambridge: Cambridge University Press; 2005.
- Wall R, Shearer D. Veterinary Entomology. 1st ed. London: Chapman & Hall Press; 1997.
- Otranto D, Lia RP, Traversa D, Giannetto S. Thelazia callipaeda (Spirurida, Thelaziidae) of carnivores and humans: morphological study by light and scanning electron microscopy. Parassitologia. 2003;45:125–33.
- Oliver JH. Importance of systematics to public health: ticks, microbes, and disease. Ann Mo Bot Gard. 1996;83:37–46.
- Whiting MF, Whiting AS, Hastriter MW, Dittmar K. A molecular phylogeny of fleas (Insecta: Siphonaptera): origins and host associations. Cladistics. 2008;24:677–707.
- Irwin PJ, Jefferies R. Arthropod-transmitted diseases of companion animals in Southeast Asia. Trends Parasitol. 2004;20:27–34.
- Nava S, Mastropaolo M, Venzal JM, Mangold AJ, Guglielmone AA. Mitochondrial DNA analysis of Rhipicephalus sanguineus sensu lato (Acari: Ixodidae) in the Southern Cone of South America. Vet Parasitol. 2012;190:547–55.
- Dantas-Torres F, Latrofa MS, Annoscia G, Giannelli A, Parisi A, Otranto D. Morphological and genetic diversity of Rhipicephalus sanguineus sensu lato from the New and Old Worlds. Parasit Vectors. 2013;6:213.
- Nava S, Beati L, Venzal JM, Labruna MB, Szabó MPJ, Petney T, et al. Rhipicephalus sanguineus (Latreille, 1806): Neotype designation, morphological re-description of all parasitic stages and molecular characterization. Ticks Tick Borne Dis. 2018;9:1573–85.
- Petney TN, Keirans JE. Ticks of the genera Boophilus, Dermacentor, Nosomma and Rhipicephalus (Acari: Ixodidae) in South-east Asia. Trop Biomed. 1996;13:73–84.
- Inpankaew T, Hii SF, Chimnoi W, Traub RJ. Canine vector-borne pathogens in semi-domesticated dogs residing in northern Cambodia. Parasit Vectors. 2016;9:253.
- Khoo JJ, Lim FS, Tan KK, Chen FS, Phoon WH, Khor CS, et al. Detection in Malaysia of a Borrelia sp. From Haemaphysalis hystricis (Ixodida: Ixodidae). J Med Entomol. 2017;54:1444–8.
- Hii SF, Lawrence AL, Cuttell L, Tynas R, Abd Rani PA, Šlapeta J, et al. Evidence for a specific host-endosymbiont relationship between ‘Rickettsia sp. genotype RF2125’ and Ctenocephalides felis orientis infesting dogs in India. Parasit Vectors. 2015;8:169.
- Kho KL, Tay ST. Identification of rickettsial infections (Rickettsia sp. TH2014) in Ctenocephalides orientis fleas (Siphonaptera: Pulicidae). J Med Entomol. 2019;56:526–32.
- Lawrence AL, Webb CE, Clark NJ, Halajian A, Mihalca AD, Miret J, et al. Out-of-Africa, human-mediated dispersal of the common cat flea, Ctenocephalides felis: The hitchhiker’s guide to world domination. Int J Parasitol. 2019;49:321–36.
- Seyyed-Zadeh SJ, Bozorg-Omid F, Telmadarraiy Z, Terenius O, Chavshin AR. Evidence for the presence of Ctenocephalides orientis in livestock dwellings in northwest Iran. Med Vet Entomol. 2018;32:383–7.
- United Nations Development Programme. Human development indices and indicators: 2018 statistical update [cited 2019 Dec 28].
- Foley RH. Parasitic mites of dogs and cats. Compend Contin Educ Vet Med. 1991;13:783–800.
- Tan LH, Fong MY, Mahmud R, Muslim A, Lau YL, Kamarulzaman A. Zoonotic Brugia pahangi filariasis in a suburbia of Kuala Lumpur City, Malaysia. Parasitol Int. 2011;60:111–3.
- Ramaiah KD, Ottesen EA. Progress and impact of 13 years of the global programme to eliminate lymphatic filariasis on reducing the burden of filarial disease. PLoS Negl Trop Dis. 2014;8:
e3319 . - Centers for Disease Control and Prevention. Dirofliariasis FAQs [cited 2019 Dec 28].
- Specht S, Suma TK, Pedrique B, Hoerauf A. Elimination of lymphatic filariasis in South East Asia. BMJ. 2019;364:k5198.
- Mallawarachchi CH, Nilmini Chandrasena TGA, Premaratna R, Mallawarachchi SMNSM, de Silva NR. Human infection with sub-periodic Brugia spp. in Gampaha District, Sri Lanka: a threat to filariasis elimination status? Parasit Vectors. 2018;11:68.
- Guerin PJ, Olliaro P, Sundar S, Boelaert M, Croft SL, Desjeux P, et al. Visceral leishmaniasis: current status of control, diagnosis, and treatment, and a proposed research and development agenda. Lancet Infect Dis. 2002;2:494–501.
- Leelayoova S, Siripattanapipong S, Manomat J, Piyaraj P, Tan-Ariya P, Bualert L, et al. Leishmaniasis in Thailand: a review of causative agents and situations. Am J Trop Med Hyg. 2017;96:534–42.
- Chusri S, Thammapalo S, Chusri S, Thammapalo S, Silpapojakul K, Siriyasatien P. Animal reservoirs and potential vectors of Leishmania siamensis in southern Thailand. Southeast Asian J Trop Med Public Health. 2014;45:13–9.
- Otranto D, Dantas-Torres F. The prevention of canine leishmaniasis and its impact on public health. Trends Parasitol. 2013;29:339–45.
- Huggins LG, Koehler AV, Ng-Nguyen D, Wilcox S, Schunack B, Inpankaew T, et al. A novel metabarcoding diagnostic tool to explore protozoan haemoparasite diversity in mammals: a proof-of-concept study using canines from the tropics. Sci Rep. 2019;9:12644.
- Nguyen VL, Colella V, Iatta R, Bui KL, Dantas-Torres F, Otranto D. Ticks and associated pathogens from dogs in northern Vietnam. Parasitol Res. 2019;118:139–42.
Figures
Tables
Cite This ArticleOriginal Publication Date: May 07, 2020
No hay comentarios:
Publicar un comentario