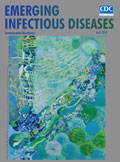
Volume 24, Number 4—April 2018
Research
Artemisinin-Resistant Plasmodium falciparum with High Survival Rates, Uganda, 2014–2016
On This Page
Mie Ikeda, Megumi Kaneko, Shin-Ichiro Tachibana, Betty Balikagala, Miki Sakurai-Yatsushiro, Shouki Yatsushiro, Nobuyuki Takahashi, Masato Yamauchi, Makoto Sekihara, Muneaki Hashimoto1, Osbert T. Katuro2, Alex Olia3, Paul S. Obwoya, Mary A. Auma, Denis A. Anywar, Emmanuel I. Odongo-Aginya, Joseph Okello-Onen4, Makoto Hirai, Jun Ohashi, Nirianne M.Q. Palacpac, Masatoshi Kataoka, Takafumi Tsuboi, Eisaku Kimura, Toshihiro Horii, and Toshihiro Mita

Abstract
Because ≈90% of malaria cases occur in Africa, emergence of artemisinin-resistant Plasmodium falciparum in Africa poses a serious public health threat. To assess emergence of artemisinin-resistant parasites in Uganda during 2014–2016, we used the recently developed ex vivo ring-stage survival assay, which estimates ring-stage–specific P. falciparum susceptibility to artemisinin. We conducted 4 cross-sectional surveys to assess artemisinin sensitivity in Gulu, Uganda. Among 194 isolates, survival rates (ratio of viable drug-exposed parasites to drug-nonexposed controls) were high (>10%) for 4 isolates. Similar rates have been closely associated with delayed parasite clearance after drug treatment and are considered to be a proxy for the artemisinin-resistant phenotype. Of these, the PfKelch13 mutation was observed in only 1 isolate, A675V. Population genetics analysis suggested that these possibly artemisinin-resistant isolates originated in Africa. Large-scale surveillance of possibly artemisinin-resistant parasites in Africa would provide useful information about treatment outcomes and help regional malaria control.
Despite reports of decreasing incidence and deaths from malaria, this disease remains a global health problem; in 2016, an estimated 216 million new cases and 445,000 deaths occurred (1). One of the main causes for the reduction of the malaria burden is the global deployment of artemisinin-based combination therapies as a first-line treatment (1). However, since first reported in 2007–2008 (2), artemisinin-resistant Plasmodium falciparumparasites have spread into the Greater Mekong Subregion of Southeast Asia (3,4). In western Cambodia, recent increases in the prevalence of piperaquine-resistant parasites have further reduced effectiveness of dihydroartemisinin/piperaquine (5).
Until now, there was no clear evidence for the emergence of P. falciparum artemisinin-resistant isolates in Africa (6). The standard for monitoring the emergence of artemisinin resistance has been the effectiveness of artemisinin-based combination therapies. In high-transmission regions, as in many parts of Africa where most persons have some immunity to malaria, partially immune persons may, however, experience some response to drug treatment even if they are infected by drug-resistant parasites (7,8). Interpretations of drug effectiveness could also be made difficult with the widely used ex vivo conventional drug-susceptibility assay (3,9,10) because reduced susceptibility to artemisinin is limited to the very narrow early ring stage of the parasites (10–12).
The recently developed ring-stage survival assay (RSA) can evaluate ring-stage–specific reduction of artemisinin susceptibility (12). In this assay, ring-stage parasites are exposed to 700 nmol/L of dihydroartemisinin for 6 h and then cultured for 66 h in the absence of the drug. Levels of artemisinin susceptibility are estimated according to the survival rate (i.e., the ratio of viable parasites exposed to 700 nmol/L dihydroartemisinin to parasites cultured simultaneously in drug-free medium). In a study of isolates from 31 persons in Cambodia, all isolates classified as artemisinin resistant by ex vivo conventional assay (having parasite clearance half-lives >5 h after artemisinin treatment [4,13]), also had an ex vivo survival rate >10% by RSA (12).
In Africa, few attempts have been made to detect artemisinin-resistant parasites by using ex vivo RSA. So far, only 2 studies have been reported, both of which showed the absence of P. falciparumisolates with survival rates >10% (14,15). Close monitoring is nevertheless valuable because parasites resistant to all widely used antimalarial drugs (chloroquine [16], pyrimethamine [17], and sulfadoxine [18]) have migrated from Southeast Asia to sub-Saharan Africa. Also reported in Africa is indigenous emergence of parasites, albeit all of minor lineages, resistant to these antimalarial drugs (19,20).
During 2014–2016, to assess artemisinin sensitivity in Gulu, northern Uganda, we performed 4 cross-sectional surveys. We evaluated the emergence of artemisinin resistance by using 3 methods: ex vivo RSA for dihydroartemisinin; conventional ex vivo drug susceptibility assay; and genotyping of PfKelch13, the gene responsible for artemisinin resistance in Southeast Asia (21–24).
Study Site, Design, and Patients
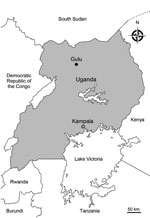
Figure 1. Site of study of ex vivo ring-stage Plasmodium falciparum survival rates, Gulu (black circle), northern Uganda, 2014–2016.
During 2014–2016, we conducted 4 cross-sectional surveys in Gulu, northern Uganda (Figure 1). In this region, malaria transmission is high; estimated prevalence is ≈60%. Each year, weak seasonal fluctuation is observed; peaks occur in May and October. All 4 surveys were performed during peak malaria seasons: October–November 2014, May–June 2015, October–November 2015, and June–July 2016. The major mosquito vector in this region is Anopheles funestus (25), and the entomological inoculation rate was estimated at >100 infective mosquito bites/person/year (26). Since 2004, artemether/lumefantrine has been used as a first-line treatment for uncomplicated malaria (27). Persons who visited St. Mary’s Hospital Lacor, Gulu, Uganda, with signs and symptoms suggestive of malaria were screened by microscopic examination of Giemsa-stained blood smears. We enrolled those with P. falciparum monoinfection (parasitemia >0.1%) if written informed consent was obtained from a parent/guardian (for patients <7 years of age), from a parent/guardian and from the patient (for patients 7–17 years of age), or from adult patients (>18 years of age). Ethics approval for the study was obtained from the Lacor Hospital Institutional Research and Ethics Committee (LH 021/09/13), the Ugandan National Council for Science and Technology (HS 1395), and the institutional review board at Juntendo University in Tokyo, Japan (2014168).
Ex Vivo RSA
We performed the ex vivo RSA as described previously, with some modifications (12,28). In brief, 1 mL of venous blood was immediately transferred to the hospital laboratory. After plasma removal, erythrocyte pellets were washed 3 times in RPMI-1640 (Thermo Fisher Scientific, Waltham, MA, USA) with 100 μg/mL gentamicin. Washed infected erythrocytes were suspended in RPMI-1640, 25 mM HEPES (4-[2-hydroxyethyl]-1-piperazineethanesulfonic acid), 2 mM l -glutamine supplemented with 100 μg/mL gentamicin and heat-inactivated 10% serum type AB from Japanese volunteers, and stored at 4°C before initiation of the ex vivo RSA. All procedures were performed within 4 h after blood collection.
The next morning, 100 μL/well of parasite culture mixture adjusted to 2% hematocrit was dispensed with 700 nmol/L dihydroartemisinin (Tokyo Chemical Industry Co. Ltd, Tokyo, Japan). As nonexposed control, 0.1% DMSO (dimethyl sulfoxide) was used. Parasitemia >1% was adjusted to 1% by adding uninfected O-type erythrocytes. Cultures were incubated in a gas atmosphere with 5% CO2 and 5% O2 by using the AnaeroPack malaria culture system (Mitsubishi Gas Chemical Co. Inc., Tokyo, Japan). After 6 h of exposure to dihydroartemisinin, pellets were washed 3 times in RPMI-1640 with gentamicin, resuspended in fresh culture medium, and incubated for another 66 h. Assays were performed in duplicate for all samples except those obtained October–November 2014 because of insufficient sample volume.
At the end of the culture period, by counting 20,000 erythrocytes and using thin blood smears, 2 investigators independently determined the number of viable parasites with normal morphologic appearance that developed into ring stages, trophozoites, and schizonts. Parasites that appeared pyknotic were considered to be dead (28,29). When the proportion of viable parasites in the nonexposed culture at 72 h was higher than the initial parasitemia at 0 h, the samples were considered to be interpretable (29). The investigators calculated survival rates as the ratios of parasites in exposed and nonexposed cultures (12); if the survival rates calculated by 2 researchers differed by >50%, a third researcher assessed the slides. Based on previous comparative studies of ex vivo RSA and in vivo drug susceptibility tests (12), the cutoff for a high survival rate by RSA was >10%. This cutoff has 89% sensitivity and 91% specificity in predicting parasites likely to have >5 h of clearance half-life after artemisinin treatment. As a reference for ex vivo RSA, we used laboratory-adapted artemisinin-susceptible (3D7) and artemisinin-resistant clones (MRA-1236 and MRA-1240) (12). The P. falciparum artemisinin-resistant strains IPC 3445 Pailin Cambodia 2010 (MRA-1236) and IPC 5202 Battanbang Cambodia 2011 (MRA-1240) were contributed by Didier Ménard and provided by the Malaria Research and Reference Reagent Resource Center (part of BEI Resources, National Institute of Allergy and Infectious Diseases, National Institutes of Health, Rockville, MD, USA).
Ex Vivo Conventional Drug-Susceptibility Assay
For each sample, 100 μL of parasite culture with parasitemia adjusted to 0.05% at 2.5% hematocrit was pipetted per well into predosed culture plates and incubated under the same conditions as the ex vivo RSA for 72 h. Wells A–H were dosed with 0 (control), 0.25, 0.5, 1, 2, 4, 8, or 16 nmol/L of dihydroartemisinin, respectively. Samples were then frozen (−20°C overnight) and thawed until complete hemolysis was obtained. We assessed parasite growth by using an ELISA that quantifies parasite histidine-rich protein 2, as reported previously (30). We established the effective concentration of dihydroartemisinin needed to inhibit growth of P. falciparum by 50% (IC50) by nonlinear regression in the online ICEstimator software (http://www.antimalarial-icestimator.net) (31).
Genotyping
We extracted parasite DNA from blood-spotted filter paper (ET31CHR; Whatman Limited, Kent, UK) by using QIAcube (QIAGEN, Hilden, Germany). The sequence of 6 propeller domains in PfKelch13 was determined as previously reported (32). From isolates showing high RSA rates of survival, we determined the following 6 mutations suggested as background genetic changes for artemisinin resistance as described (32,33): D193Y in ferredoxin (fd), T484I in multidrug resistance protein 2 (mdr2), V127M in apicoplast ribosomal protein S10 (arps10), I356T in chloroquine resistance transporter (crt), V1157L in protein phosphatase (pph), and C1484F in phosphoinositide-binding protein (pibp). Samples with minor peaks >50% the height of the major peak were considered mixed genotypes.
Whole-Genome Sequencing and Variant Detection
For 3 isolates with high survival rates by RSA, we extracted genomic DNA from leukocyte-removed blood with Acrodisc filters (Pall Corporation, New York, NY, USA) by using a QIAamp DNA Blood Mini Kit (QIAGEN). We prepared DNA libraries by using a TruSeq DNA PCR-Free Library Preparation Kit (Illumina, San Diego, CA, USA) after amplification of the genomic DNA with an Illustra GenomiPhi DNA Amplification Kit (GE Healthcare, Chicago, IL, USA) or without genome amplification with a Nextera XT DNA Sample Prep Kit (Illumina). We obtained ≈1–1.5 Gb of data per sample, consisting of paired-end reads 100 or 150 bp long by using Illumina instruments (Miseq and Hiseq2000). Data are available at the DDBJ Sequence Read Archive (http://trace.ddbj.nig.ac.jp/dra/index.html) under accession nos. DRA005346, DRA005347, and DRA005348.
For comparative analyses, we obtained raw sequence data (fastq files) of 31 P. falciparum isolates from various regions in Asia (n = 19) and Africa (n = 12) from the Short Read Archive at the National Center for Biotechnology Information (https://www.ncbi.nlm.nih.gov/sra) (Technical Appendix[PDF - 251 KB - 3 pages] Table 1). Among 19 isolates from Asia, 6 harbored mutant alleles in PfKelch13. We mapped all short-read data onto the 3D7 reference genome (National Center for Biotechnology Information BioProject ID PRJNA148) by using CLC Genomics Workbench software (QIAGEN) with default parameters. We then used the resulting files for detecting single-nucleotide polymorphisms (SNPs) by using the Basic Variant Detection program in CLC Genomics Workbench. SNPs were called at all genomic positions with >80% frequency of >10 reads support.
Population Genetic Analyses
To clarify lineages of isolates exhibiting high rates of survival according to RSA, we performed principal component analysis and STRUCTURE analysis (34). SNPs with minor allele frequency >25% were used after standardization. For principal component analysis, we used BellCurve for Excel (Social Survey Research Information Co., Ltd, Tokyo, Japan). We used STRUCTURE 2.3.3 to assign individual isolates from all populations to 2 clusters (i.e., K = 2). For each run, a burn-in period of 10,000 steps was followed by 10,000 iterations under the admixture model and the assumption of uncorrelated haplotype frequencies among populations.
Ex Vivo RSA
In the ex vivo RSA study, we enrolled 249 patients (Table 1). Most patients were children; median age was 3 years. Fever >37.5°C was reported for 83% of patients. Other signs and symptoms were present for 7%–22% of enrolled patients. Examination of blood smears and species-specific PCR revealed that all blood samples contained monoinfections with P. falciparum.
We obtained successful ex vivo RSA results for dihydroartemisinin for 194 (77.9%) patients. Unsuccessful results were mostly caused by insufficient parasite growth (n = 28) or inability to count 20,000 erythrocytes because of low-quality blood smears and limited amounts of blood (n = 27). We found no significant differences for patient background characteristics or parasitemia in all enrolled and ex vivo RSA–successful patients (Table 1; Technical Appendix[PDF - 251 KB - 3 pages] Table 2). Artemisinin-susceptible laboratory clone 3D7 showed no parasites at 700 nmol/L, whereas artemisinin-resistant laboratory clones MRA-1236 and MRA-1240 showed survival rates (± SEM) of >10% (14.3 ± 0.5% and 27.0 ± 2.7%, respectively) (Table 2).
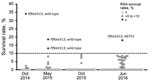
Figure 2. Distribution of ex vivo ring-stage Plasmodium falciparum survival rates, by RSA during each study period, 2014–2016. Susceptibility to dihydroartemisinin was determined by using ex vivo RSA survival rates. Survival rate was...
Among the study samples, 4 (2.1%) were classified as having high parasite survival rates by RSA; mean survival rates (± SEM) ranged from 13.3% ± 1.5% (isolate H2) to 34.3% (isolate H1) (Figure 2, Table 2). We assayed all except 1 isolate (H1) in duplicate. We observed these resistant isolates in all sampling periods except October–November 2015. One isolate (H1) had higher survival rates (34.3%) than the 2 artemisinin-resistant laboratory clones from Cambodia (MRA-1236 and MRA-1240). Developmental stages of the parasites at enrollment were microscopically determined (Technical Appendix[PDF - 251 KB - 3 pages] Figure). In the 3 isolates with high survival rates by RSA, proportions of early ring-stage parasites at enrollment were high (72%–98%) (Technical Appendix[PDF - 251 KB - 3 pages] Table 3), except for 1 isolate (H3), which had a lower proportion of early ring-stage parasites. For parasites with survival rates ≈10% (I1, I5, and I7), early ring-stage parasites were ≈51.9%–57.7% and the rest were late ring-stage parasites. These observations show that the developmental stages of the parasite before start of the RSA was not biased or skewed so as to influence RSA results. In fact, survival rates of samples with an almost 50:50 ratio of parasites in early and late stages might be underestimated because susceptibility to artemisinin occurs only during the early ring stage (10–12).
Conventional Ex Vivo Drug Susceptibility Assay for Dihydroartemisinin
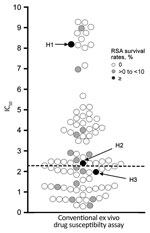
Figure 3. Distribution of IC50 of Plasmodium falciparum values by dihydroartemisinin in conventional ex vivo drug susceptibility assay. IC50values to dihydroartemisinin in conventional ex vivo drug susceptibility assay are plotted. Geometric mean...
We performed a conventional ex vivo dihydroartemisinin-susceptibility assay in all but 1 survey (June–July 2016) and successfully determined ex vivo RSA and conventional ex vivo IC50 values for 93 patients (Figure 3). IC50 values were not correlated with survival rates by RSA. Geometric mean IC50 for isolates with high survival rates by RSA (3.3 nmol/L) were similar to those for the artemisinin-susceptible isolates (2.25 nmol/L).
Genotypes of PfKelch13 and Potential Background Mutations
Only 5 (2.6%) isolates (C469Y, M472V, A621S, V666I, A675V) harbored nonsynonymous mutations at propeller domains in PfKelch13, all of which were observed as singletons (Technical Appendix[PDF - 251 KB - 3 pages] Table 4). Among 4 parasites with high survival rates by RSA, 1 harbored the A675V mutation and the other 3 harbored the wild-type allele (Figure 2). We observed no background genetic changes for artemisinin resistance (D193Y in fd, T484I in mdr2, V127M in arps10, I356T in crt, V1157L in pph, and C1484F in pibp; data not shown).
Lineages of Parasites with High Survival Rates by RSA
To clarify whether parasites with high survival rates by RSA indigenously originated in Africa or migrated from Southeast Asia, we performed principal component and STRUCTURE analyses. Whole-genome sequences were determined for all except 1 (H1) parasite isolate with a high survival rate by RSA. As reference, we used 31 P. falciparum isolates with origins from Asia or Africa. High-quality whole-genome sequence data enabled identification of 174,266 SNPs, of which 168,908 were biallelic SNPs. Among these, we used 14,341 SNPs with minor allele frequency >25% for the analyses. In principal component analysis, the first principal component clearly separated isolates from Africa from those from Southeast Asia (Figure 4, panel A). All 7 reference isolates harboring mutant PfKelch13 were located in the cluster from Southeast Asia. In contrast, all 3 isolates from Uganda with high survival rates by RSA were inside the cluster from Africa. STRUCTURE analysis also clarified the distinct population structure of isolates from Southeast Asia compared with all isolates from Africa, including the 3 isolates with high survival rates by RSA (Figure 4, panel B).
Emergence and spread of artemisinin-resistant P. falciparum outside the Greater Mekong Subregion pose a serious public health threat, especially in sub-Saharan Africa, the region that in 2015 accounted for 90% of global malaria cases and 92% of malaria deaths (35). The recently developed ex vivo RSA found that 4 (2.1%) isolates from Uganda showed high (>10%) survival rates, levels of which are reported to be closely associated with slow-clearing P. falciparum infections (12). Among these, mutation in PfKelch13 was observed in only 1 isolate. Population genetic analysis with whole-genome sequences demonstrated that these isolates belong to the cluster from Africa, suggesting an indigenous origin rather than migration from Southeast Asia.
So far, 2 ex vivo RSA results have been reported in sub-Saharan Africa: in Cameroon (15) and in Kampala, Uganda, ≈300 km from our study site (14). Neither study found isolates with high survival rates. In our analysis, 4 (2.1%) isolates had survival rates >10%. In addition, 3 (1.5%) isolates with survival rates of ≈10% had lower proportions of early ring-stage parasites (51.9%–57.7%) at patient enrollment. Because artemisinin resistance is known to be confined to the early ring stage (10–12), the observed survival rate for these isolates might be underestimated. For our analysis, we assumed that an overall 2%–4% of isolates had artemisinin-resistant potential. Since the discovery of PfKelch13 as an artemisinin-resistant responsible gene in 2013 (21), numerous molecular epidemiologic studies identified >200 mutations in this gene (4,35,36). The correlation with clinical and laboratory artemisinin resistance in 6 mutations (N458Y, Y493H, R539T, I543T, R561H, C580Y) has been validated (37). In our study, 1 isolate with a high survival rate by RSA harbored a mutation allele in PfKelch13 (A675V). This mutation is reported to have a level of parasite clearance half-life similar to that of the most prevalent C580Y mutation (4,38) and has thus been regarded as candidate artemisinin-related mutation (37). The allele is distributed in Southeast Asia (Thailand–Myanmar border) and sub-Saharan Africa (Democratic Republic of the Congo and Rwanda) (4,38–40). However, our population genomic analysis revealed that this isolate belongs to African lineage, suggesting that artemisinin-resistant migration of A675V from Southeast Asia to Africa is less likely than the indigenous emergence of this mutation in Africa.
Three other isolates with high survival rates by RSA harbored a wild-type allele in PfKelch13. A similar finding in Cambodia has been recently reported (41). The parasiticidal mechanism of artemisinin is known to result from induction of highly reactive carbon-centered radicals that inactivate multiple crucial proteins (42). To combat this effect, resistant parasites are believed to enhance the oxidative stress response, altering the cell cycle (29,36,39,43–45). Recent population transcriptome analyses that used 1,043 clinical P. falciparum isolates obtained directly from patients with acute falciparum malaria demonstrated associations between PfKelch13 mutations and upregulation of unfolded protein response, one of the stress response systems, and PI3K/PI3P/AKT pathways (46). One possible hypothesis to explain the absence of a PfKelch13 mutation in the ex vivo RSA artemisinin-resistant isolates is the activation of alternate pathways by yet unknown mechanism(s).
Witkowski et al. showed a strong correlation between ex vivo RSA survival rates and in vivo parasite clearance half-lives in Cambodia (12). When the ex vivo RSA survival rate cutoff of 10% is applied to their study, 29 of 30 infections are accurately identified as artemisinin resistant and artemisinin susceptible (12). This cutoff point produced 89% sensitivity and 91% specificity for in vivo artemisinin resistance in the parasites from Cambodia (12). However, a similar correlation study is lacking for isolates from Africa. Because many factors such as levels of host immunity and pharmacokinetics could complicate drug clinical effectiveness, further correlation of ex vivo RSA and in vivo studies is strongly required in the various malaria-endemic regions with different population ethnicities and malaria ecologies.
Our study has several limitations. Parasites were not cryopreserved; thus, survival rate was not reconfirmed by in vitro RSA with culture-adapted parasites. Reconfirmation was not performed because of the difficulties in logistics and cryopreservation of natural parasites at our field site and transportation to our laboratory. However, we performed ex vivo RSA duplicate (except during October–November 2014), enabling confirmation via independently performed assays. In addition, we did not obtain clinical confirmation of artemisinin resistance for all 4 isolates with high survival rates by RSA because clinical study in 2014 was performed only during October–November, and only in 9 random isolates was artemether/lumefantrine treatment efficacy assessed.
In the conventional ex vivo artemisinin susceptibility assay, parasites are exposed to dihydroartemisinin for 48–72 h, affecting trophozoite and schizont stages. Strictly, therefore, this assay theoretically underestimates the level of artemisinin resistance. Using ex vivo assays, we found that the mean IC50 in isolates with high survival rates by RSA was similar to that for those isolates characterized as having low survival rates or those that are completely susceptible (showed no survival by RSA). This finding was similar to previous field observations in Cambodia, where a lack of correlation was found between ex vivo survival rates by RSA and IC50s to dihydroartemisinin in conventional ex vivo drug susceptibility assay (12), supporting the notion that conventional ex vivo assays may not be useful for properly distinguishing isolates with decreased artemisinin susceptibility in the field.
In conclusion, our analysis has revealed the potential emergence of P. falciparum with high survival rates by RSA in Uganda. One of these parasites harbored a PfKelch13 A675V mutation. Because ≈90% of malaria cases occur in Africa (35), emergence and spread of artemisinin resistance impose substantial obstacles for effective malaria control and the approach toward elimination. It is thus imperative to perform further intensive surveillance for artemisinin resistance in various malaria-endemic regions in Africa and to elucidate genetic changes that confer resistance to artemisinin in parasites in Africa. Use of ex vivo RSA would help solve these challenges.
Dr. Ikeda is a postdoctoral student at the Department of Tropical Medicine and Parasitology, Juntendo University. Her research interest includes epidemiology of drug-resistant malaria.
Acknowledgments
We thank the many doctors, nurses, and laboratory technicians at St. Mary’s Hospital Lacor, who greatly contributed to the hospital survey. We also thank Sakurako Emoto, Keishi Fukuhara, Nagamasa Kano, Akimasa Maeta, Akihiro Kosuge, Azusa Takahashi, Koji Ijichi, Ryoichi Matsubara, Kouki Soda, and Sachifumi Sakamoto for their support in the field.
This publication uses data from the MalariaGEN Plasmodium falciparum Community Project as described in Genomic Epidemiology of Artemisinin-Resistant Malaria, eLife, 2016 (). This work was supported by Grants-in-aid for Scientific Research (Ministry of Education, Culture, Sports, Science, and Technology of Japan) to T.M. and by funds for Integrated Promotion of Social System Reform and Research and Development (Ministry of Education, Culture, Sports, Science, and Technology of Japan), and grants from the Translational Research Network program (Japan Agency for Medical Research and Development) and the Global Health Innovative Technology Fund (G2013-105) to T.H.
References
- World Health Organization. World malaria report 2017. Geneva: The Organization; 2017.
- Noedl H, Se Y, Schaecher K, Smith BL, Socheat D, Fukuda MM; Artemisinin Resistance in Cambodia 1 (ARC1) Study Consortium. Evidence of artemisinin-resistant malaria in western Cambodia. N Engl J Med. 2008;359:2619–20. DOIPubMed
- Dondorp AM, Nosten F, Yi P, Das D, Phyo AP, Tarning J, et al. Artemisinin resistance in Plasmodium falciparum malaria. N Engl J Med. 2009;361:455–67. DOIPubMed
- Ashley EA, Dhorda M, Fairhurst RM, Amaratunga C, Lim P, Suon S, et al.; Tracking Resistance to Artemisinin Collaboration (TRAC). Spread of artemisinin resistance in Plasmodium falciparummalaria. N Engl J Med. 2014;371:411–23. DOIPubMed
- Amaratunga C, Lim P, Suon S, Sreng S, Mao S, Sopha C, et al. Dihydroartemisinin-piperaquine resistance in Plasmodium falciparum malaria in Cambodia: a multisite prospective cohort study.Lancet Infect Dis. 2016;16:357–65. DOIPubMed
- World Health Organization. World malaria report 2015. Geneva: The Organization; 2015.
- Rogerson SJ, Wijesinghe RS, Meshnick SR. Host immunity as a determinant of treatment outcome in Plasmodium falciparum malaria. Lancet Infect Dis. 2010;10:51–9. DOIPubMed
- Lopera-Mesa TM, Doumbia S, Chiang S, Zeituni AE, Konate DS, Doumbouya M, et al. Plasmodium falciparum clearance rates in response to artesunate in Malian children with malaria: effect of acquired immunity. J Infect Dis. 2013;207:1655–63. DOIPubMed
- Amaratunga C, Sreng S, Suon S, Phelps ES, Stepniewska K, Lim P, et al. Artemisinin-resistant Plasmodium falciparum in Pursat province, western Cambodia: a parasite clearance rate study.Lancet Infect Dis. 2012;12:851–8. DOIPubMed
- Klonis N, Xie SC, McCaw JM, Crespo-Ortiz MP, Zaloumis SG, Simpson JA, et al. Altered temporal response of malaria parasites determines differential sensitivity to artemisinin. Proc Natl Acad Sci U S A. 2013;110:5157–62. DOIPubMed
- Witkowski B, Lelièvre J, Barragán MJ, Laurent V, Su XZ, Berry A, et al. Increased tolerance to artemisinin in Plasmodium falciparum is mediated by a quiescence mechanism. Antimicrob Agents Chemother. 2010;54:1872–7. DOIPubMed
- Witkowski B, Amaratunga C, Khim N, Sreng S, Chim P, Kim S, et al. Novel phenotypic assays for the detection of artemisinin-resistant Plasmodium falciparum malaria in Cambodia: in-vitro and ex-vivo drug-response studies. Lancet Infect Dis. 2013;13:1043–9. DOIPubMed
- Phyo AP, Nkhoma S, Stepniewska K, Ashley EA, Nair S, McGready R, et al. Emergence of artemisinin-resistant malaria on the western border of Thailand: a longitudinal study. Lancet. 2012;379:1960–6. DOIPubMed
- Cooper RA, Conrad MD, Watson QD, Huezo SJ, Ninsiima H, Tumwebaze P, et al. Lack of artemisinin resistance in Plasmodium falciparum in Uganda based on parasitological and molecular assays. Antimicrob Agents Chemother. 2015;59:5061–4. DOIPubMed
- Menard S, Tchoufack JN, Maffo CN, Nsango SE, Iriart X, Abate L, et al. Insight into k13-propeller gene polymorphism and ex vivo DHA-response profiles from Cameroonian isolates. Malar J. 2016;15:572. DOIPubMed
- Wootton JC, Feng X, Ferdig MT, Cooper RA, Mu J, Baruch DI, et al. Genetic diversity and chloroquine selective sweeps in Plasmodium falciparum. Nature. 2002;418:320–3. DOIPubMed
- Roper C, Pearce R, Nair S, Sharp B, Nosten F, Anderson T. Intercontinental spread of pyrimethamine-resistant malaria. Science. 2004;305:1124. DOIPubMed
- Mita T, Venkatesan M, Ohashi J, Culleton R, Takahashi N, Tsukahara T, et al. Limited geographical origin and global spread of sulfadoxine-resistant dhps alleles in Plasmodium falciparumpopulations. J Infect Dis. 2011;204:1980–8. DOIPubMed
- McCollum AM, Poe AC, Hamel M, Huber C, Zhou Z, Shi YP, et al. Antifolate resistance in Plasmodium falciparum: multiple origins and identification of novel dhfr alleles. J Infect Dis. 2006;194:189–97. DOIPubMed
- Mita T, Tanabe K, Takahashi N, Culleton R, Ndounga M, Dzodzomenyo M, et al. Indigenous evolution of Plasmodium falciparum pyrimethamine resistance multiple times in Africa. J Antimicrob Chemother. 2009;63:252–5. DOIPubMed
- Ariey F, Witkowski B, Amaratunga C, Beghain J, Langlois AC, Khim N, et al. A molecular marker of artemisinin-resistant Plasmodium falciparum malaria. Nature. 2014;505:50–5. DOIPubMed
- Ghorbal M, Gorman M, Macpherson CR, Martins RM, Scherf A, Lopez-Rubio JJ. Genome editing in the human malaria parasite Plasmodium falciparum using the CRISPR-Cas9 system. Nat Biotechnol. 2014;32:819–21. DOIPubMed
- Straimer J, Gnädig NF, Witkowski B, Amaratunga C, Duru V, Ramadani AP, et al. Drug resistance. K13-propeller mutations confer artemisinin resistance in Plasmodium falciparum clinical isolates. Science. 2015;347:428–31. DOIPubMed
- Ménard D, Khim N, Beghain J, Adegnika AA, Shafiul-Alam M, Amodu O, et al.; KARMA Consortium. KARMA Consortium. A worldwide map of Plasmodium falciparum K13-propeller polymorphisms. N Engl J Med. 2016;374:2453–64. DOIPubMed
- Okello PE, Van Bortel W, Byaruhanga AM, Correwyn A, Roelants P, Talisuna A, et al. Variation in malaria transmission intensity in seven sites throughout Uganda. Am J Trop Med Hyg. 2006;75:219–25.PubMed
- Uganda Bureau of Statistics. Malaria Indicator Survey 2009 [cited 2017 Dec 15]. https://dhsprogram.com/pubs/pdf/MIS6/MIS6.pdf
- Yeka A, Gasasira A, Mpimbaza A, Achan J, Nankabirwa J, Nsobya S, et al. Malaria in Uganda: challenges to control on the long road to elimination: I. Epidemiology and current control efforts.Acta Trop. 2012;121:184–95. DOIPubMed
- Witkowski B, Menard D, Amaratunga C, Fairhurst RM. Ring-stage survival assays (RSA) to evaluate the in-vitro and ex-vivo susceptibility of Plasmodium falciparum to artemisinins [cited 2017 Dec 15]. http://www.wwarn.org/sites/default/files/attachments/procedures/INV10-Standard-Operating-Procedure-Ring-Stage-Survival-Assays-v1.2.pdf
- Dogovski C, Xie SC, Burgio G, Bridgford J, Mok S, McCaw JM, et al. Targeting the cell stress response of Plasmodium falciparum to overcome artemisinin resistance. PLoS Biol. 2015;13:e1002132. DOIPubMed
- Noedl H, Wernsdorfer WH, Miller RS, Wongsrichanalai C. Histidine-rich protein II: a novel approach to malaria drug sensitivity testing. Antimicrob Agents Chemother. 2002;46:1658–64. DOIPubMed
- Le Nagard H, Vincent C, Mentré F, Le Bras J. Online analysis of in vitro resistance to antimalarial drugs through nonlinear regression. Comput Methods Programs Biomed. 2011;104:10–8. DOIPubMed
- Balikagala B, Mita T, Ikeda M, Sakurai M, Yatsushiro S, Takahashi N, et al. Absence of in vivo selection for K13 mutations after artemether-lumefantrine treatment in Uganda. Malar J. 2017;16:23. DOIPubMed
- Miotto O, Amato R, Ashley EA, MacInnis B, Almagro-Garcia J, Amaratunga C, et al. Genetic architecture of artemisinin-resistant Plasmodium falciparum. Nat Genet. 2015;47:226–34. DOIPubMed
- Pritchard JK, Stephens M, Donnelly P. Inference of population structure using multilocus genotype data. Genetics. 2000;155:945–59.PubMed
- World Health Organization. World malaria report 2016. Geneva: The Organization; 2016.
- Fairhurst RM, Dondorp AM. Artemisinin-resistant Plasmodium falciparum malaria. Microbiol Spectr. 2016;4.
- World Health Organization. Artemisinin and artemisinin-based combination therapy resistance. Status report. Geneva: The Organization; 2016.
- Boullé M, Witkowski B, Duru V, Sriprawat K, Nair SK, McDew-White M, et al. Artemisinin-resistant Plasmodium falciparum K13 mutant alleles, Thailand–Myanmar border. Emerg Infect Dis. 2016;22:1503–5. DOIPubMed
- Hott A, Casandra D, Sparks KN, Morton LC, Castanares GG, Rutter A, et al. Artemisinin-resistant Plasmodium falciparum parasites exhibit altered patterns of development in infected erythrocytes. Antimicrob Agents Chemother. 2015;59:3156–67. DOIPubMed
- Tacoli C, Gai PP, Bayingana C, Sifft K, Geus D, Ndoli J, et al. Artemisinin resistance-associated K13 polymorphisms of Plasmodium falciparum in southern Rwanda, 2010–2015. Am J Trop Med Hyg. 2016;95:1090–3. DOIPubMed
- Mukherjee A, Bopp S, Magistrado P, Wong W, Daniels R, Demas A, et al. Artemisinin resistance without pfkelch13 mutations in Plasmodium falciparum isolates from Cambodia. Malar J. 2017;16:195. DOIPubMed
- O’Neill PM, Barton VE, Ward SA. The molecular mechanism of action of artemisinin—the debate continues. Molecules. 2010;15:1705–21. DOIPubMed
- Mbengue A, Bhattacharjee S, Pandharkar T, Liu H, Estiu G, Stahelin RV, et al. A molecular mechanism of artemisinin resistance in Plasmodium falciparum malaria. Nature. 2015;520:683–7. DOIPubMed
- Mita T, Tachibana S, Hashimoto M, Hirai M. Plasmodium falciparum kelch 13: a potential molecular marker for tackling artemisinin-resistant malaria parasites. Expert Rev Anti Infect Ther. 2016;14:125–35. DOIPubMed
- Paloque L, Ramadani AP, Mercereau-Puijalon O, Augereau JM, Benoit-Vical F. Plasmodium falciparum: multifaceted resistance to artemisinins. Malar J. 2016;15:149. DOIPubMed
- Mok S, Ashley EA, Ferreira PE, Zhu L, Lin Z, Yeo T, et al. Drug resistance. Population transcriptomics of human malaria parasites reveals the mechanism of artemisinin resistance. Science. 2015;347:431–5. DOIPubMed
Figures
Tables
Technical Appendix
Cite This Article1Current affiliation: National Institute of Advanced Industrial Science and Technology, Kagawa, Japan.
2Current affiliation: Mildmay Uganda-KAMPALA, Kampala, Uganda.
3Current affiliation: Gunma University, Gunma, Japan.
4Deceased.
No hay comentarios:
Publicar un comentario