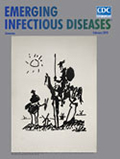
Volume 24, Number 2—February 2018
Dispatch
Effects of Culling on Leptospira interrogans Carriage by Rats
On This Page
Michael J. Lee
, Kaylee A. Byers, Christina M. Donovan, Julie J. Bidulka, Craig Stephen, David M. Patrick, and Chelsea G. Himsworth

Abstract
We found that lethal, urban rat control is associated with a significant increase in the odds that surviving rats carry Leptospira interrogans. Our results suggest that human interventions have the potential to affect and even increase the prevalence of zoonotic pathogens within rat populations.
Norway rats (Rattus norvegicus) are a reservoir for Leptospira interrogans, the etiologic agent of the zoonotic disease leptospirosis (1). Leptospirosis affects ≈1 million persons worldwide annually and can result in kidney failure or pulmonary hemorrhage (1,2). Increasing urbanization has driven the emergence of leptospirosis in cities globally (3). Within cities, areas of poverty experience a confluence of environmental and socioeconomic factors that heighten the risk for ratborne L. interrogans transmission (3).
The ecology of rats and the epidemiology of L. interrogans within their populations are intimately connected (4). Previous research on other reservoir species suggests that anthropogenic disturbances may alter reservoir ecology, resulting in new transmission patterns (5,6). Because lethal control is a common technique used to address rat populations (7,8), we aimed to determine whether culling effects L. interrogans carriage by urban Norway rats.
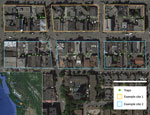
Figure 1. Two example sites side-by-side in a study of the effects of culling on Leptospira interrogans carriage by rats, Vancouver, British Columbia, Canada, June 2016–January 2017. Each site comprised 3 city blocks...
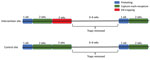
Figure 2. Experiment timeline in intervention and control sites in a study of the effects of culling on Leptospira interrogans carriage by rats, Vancouver, British Columbia, Canada, June 2016–January 2017. Trapping in each...
This study, conducted in an inner-city neighborhood of Vancouver, British Columbia, Canada, during June 2016–January 2017, compared the prevalence of L. interrogans in rat populations before and after a kill-trapping intervention. Each study site (12 total) comprised 3 contiguous city blocks and was designated as a control site or an intervention site (Figure 1). In control sites, no kill-trapping occurred; in intervention sites, kill-trapping occurred only in the central blocks, and the 2 adjacent blocks were designated as nonkill flanking blocks. We divided trapping in each intervention site into 3 time periods: before, during, and after the intervention (Figure 2). Before and after the intervention, rats were trapped, processed, and released. During processing, rats were marked with an ear tag, and morphometric information was recorded (Table 1). Urine was obtained from these rats and tested for L. interrogans by real-time PCR. During the intervention, we euthanized trapped rats; in control sites and flanking blocks, capture-release continued, and rats were not euthanized. The University of British Columbia’s Animal Care Committee (A14-0265) approved all procedures (Technical Appendix[PDF - 301 KB - 4 pages]).
We used mixed-effects multivariable logistic regression to estimate the effect of the intervention on the odds that rats carried L. interrogans, while controlling for clustering by city block (4). The outcome was the L. interrogans PCR status (negative or positive) of individual rats. The predictor variable categorized rats by block and period of capture: 0, rats caught before the intervention; 1, rats caught after the intervention in control blocks; 2, rats caught after the intervention in nonkill flanking blocks; and 3, rats caught after the intervention in intervention blocks. Although we did not undertake the intervention in control sites, we considered the third 2-week trapping period independently from the other trapping periods in control sites to detect any temporal changes in L. interrogans prevalence not associated with the intervention. We excluded the 7 rats captured both before and after the intervention to avoid double-counting individual rats. For rats recaptured within the same period as their first capture (either before or after the intervention), we averaged weight and length across captures. We also excluded 1 rat missing data for covariates under consideration.
We used a hypothesis-testing model-building approach to estimate the effect of the intervention while controlling for covariates (Table 1). We kept covariates, selected on the basis of their potential to confound the relationship between the intervention and L. interrogans carriage, in the model if they changed the estimated relationship between the predictor and outcome variables by >10%. Because length and weight were collinear, we used the covariate with the largest effect on the relationship between the predictor and outcome. We dichotomized weight around its median because it was not linear with the log-odds of the outcome. For statistical analyses, we used RStudio (Boston, MA, USA).
Of the 438 rats trapped, we included 430 in the modeling process (Table 1). Sixty-four (14.9%; 95% CI 11.7%–18.7%) rats were PCR-positive for L. interrogans. Of 131 rats recaptured, 5 were L. interrogans positive at their first capture and recapture; no positive rats changed pathogen status within a trapping period.
Rats caught in intervention blocks after an intervention had 9.55 times the odds of carrying L. interrogans than did rats trapped before an intervention, while adjusting for weight and wound presence variables (Table 2). We found no significant changes in either flanking blocks or control blocks. In this model, 52.6% of the total model variance was due to the random effect of the block (11). Rerunning the final model including animals that were caught both before and after the intervention did not substantially affect the results (effect of the intervention in intervention blocks; adjusted odds ratio 8.88, 95% CI 1.68–68.08).
This study showed that kill-trapping was associated with increased odds that rats carried L. interrogans in the city blocks where kill-trapping occurred. We did not observe this effect in control blocks or nonkill flanking blocks.
Increased intraspecific transmission of L. interrogans resulting from kill-trapping is a plausible explanation for the observed effect. Previous research suggests that rat-to-rat transmission of L. interrogans is associated with social structures in rat colonies (4). Given that culling is ineffective at removing entire rat populations (7,8,12), kill-trapping may have disrupted social structures and promoted new interactions that facilitated transmission among remaining rats. For example, culling may have removed dominant rats (13), subsequently increasing aggressive interactions among the remaining rats as they established a new social hierarchy. The positive association between L. interrogans status and weight/wound presence (which are correlated with hierarchical dominance) supports this hypothesis because the bacteria may be transmitted through specific aggressive/dominance interactions (4).
We assessed only the effect of culling on a single ratborne pathogen. L. interrogans might be particularly susceptible to the effects of culling because of its dependence on rat social structures. Other vectorborne (e.g., fleaborne Rickettsia spp. [14]) or environmentally acquired (e.g., methicillin-resistant Staphylococcus aureus [15]) rat-associated pathogens might not be as easily influenced by culling. Future studies should determine the duration of effects induced by lethal control because effects on L. interrogans prevalence may wane with time. However, given that such methods are ineffective at removing entire rat populations and might therefore be used repeatedly as the population rebounds (7,8,12), short-term effects may be particularly important.
We demonstrated that rat culling has the potential to increase the odds for L. interrogans carriage among remaining rats and thus could potentially increase the risk for transmission to humans. Although public health risks resulting from such an increase postintervention might be offset by a decrease in the number of rats, we were unable to quantify the size of the rat population before and after intervention. Practical and ethical considerations make it difficult to empirically demonstrate a direct link between culling and increased pathogen transmission from rats to humans. Rather, after culling, the potential for a person to encounter a rat carrying L. interrogans increases if a person encounters a rat, suggesting that the risk for zoonotic transmission increases per rat contact.
The convergence of this study with previous literature documenting that reactive culling is often unsuccessful at removing rat populations (7,8,12) indicates that such methods are ineffective. Instead, ecologically based rodent management, which focuses on reducing resources available to rats (8), should be more widely applied to urban environments.
By integrating our results with other studies on the impacts of culling wild animals to control communicable diseases (5,6), we can conclude that killing animal reservoirs of human pathogens might have unintended consequences on the disease risks. This hypothesis underscores the importance of understanding the ecology of the targeted animal reservoir to design effective control programs.
Mr. Lee is a PhD student in the School of Population and Public Health, University of British Columbia, in Vancouver. His research interests include the changing epidemiology of ratborne and other zoonotic pathogens.
Acknowledgments
We thank Charles Krebs, Bobby Corrigan, and Michael Whitlock for their suggestions in designing this study. We also thank Geoffrey Knaub and Sophia Kontou for their help during the field trial, as well as Erin Zabek for her assistance throughout this study in acquiring laboratory equipment and supplies. We thank the Vancouver Area Network of Drug Users for their participation in this project.
This study was funded in part by the Public Scholars Initiative at the University of British Columbia (K.A.B.), the Northwest Scientific Association (M.J.L.), and a Natural Sciences and Engineering Research Council of Canada Discovery Grant (D.M.P.).
References
- Guerra MA. Leptospirosis. J Am Vet Med Assoc. 2009;234:472–8, 430.
- Costa F, Hagan JE, Calcagno J, Kane M, Torgerson P, Martinez-Silveira MS, et al. Global morbidity and mortality of leptospirosis: a systematic review.PLoS Negl Trop Dis. 2015;9:e0003898–19. DOIPubMed
- Himsworth CG, Parsons KL, Jardine C, Patrick DM. Rats, cities, people, and pathogens: a systematic review and narrative synthesis of literature regarding the ecology of rat-associated zoonoses in urban centers. Vector Borne Zoonotic Dis. 2013;13:349–59. DOIPubMed
- Himsworth CG, Bidulka J, Parsons KL, Feng AYT, Tang P, Jardine CM, et al. Ecology of Leptospira interrogans in Norway rats (Rattus norvegicus) in an inner-city neighborhood of Vancouver, Canada. PLoS Negl Trop Dis. 2013;7:e2270–9. DOIPubMed
- Amman BR, Nyakarahuka L, McElroy AK, Dodd KA, Sealy TK, Schuh AJ, et al. Marburgvirus resurgence in Kitaka Mine bat population after extermination attempts, Uganda. Emerg Infect Dis. 2014;20:1761–4. DOIPubMed
- Donnelly CA, Woodroffe R, Cox DR, Bourne FJ, Cheeseman CL, Clifton-Hadley RS, et al. Positive and negative effects of widespread badger culling on tuberculosis in cattle. Nature. 2006;439:843–6. DOIPubMed
- Meyer A. Urban commensal rodent control: fact or fiction? In: Singleton GR, Hinds LA, Krebs CJ, Spratt DM, editors. ACIAR monograph series. Canberra (ACT, Australia): Australian Centre for International Agricultural Research; 2003. p. 446–50.
- Colvin BA, Jackson WB. Urban rodent control programs for the 21st century. In: Singleton GR, Hinds LA, Leirs H, Zhang Z, editors. ACIAR monograph series. Canberra (ACT, Australia): Australian Centre for International Agricultural Research; 1999. p. 243–57.
- Davis DE, Emlen JT, Stokes AW. Studies on home range in the brown rat. J Mammal. 1948;29:207–25. DOI
- Gardner-Santana LC, Norris DE, Fornadel CM, Hinson ER, Klein SL, Glass GE. Commensal ecology, urban landscapes, and their influence on the genetic characteristics of city-dwelling Norway rats (Rattus norvegicus). Mol Ecol. 2009;18:2766–78. DOIPubMed
- Dohoo I, Martin W, Stryhn H. Veterinary epidemiological research. Charlottetown (PEI, Canada): AVC Inc.; 2007.
- Cowan DP, Quy RJ, Lambert MS. Ecological perspectives on the management of commensal rodents. In: Singleton GR, Hinds LA, Krebs CJ, Spratt DM, editors. ACIAR Monograph series. Canberra (ACT, Australia): Australian Centre for International Agricultural Research; 2003. p. 433–39.
- Clapperton BK. A review of the current knowledge of rodent behaviour in relation to control devices. Science for conservation monograph series. Wellington (New Zealand): Science & Technical Publishing, New Zealand Department of Conservation; 2006.
- Himsworth CG, Bai Y, Kosoy MY, Wood H, DiBernardo A, Lindsay R, et al. An investigation of Bartonella spp., Rickettsia typhi, and Seoul hantavirus in rats (Rattus spp.) from an inner-city neighborhood of Vancouver, Canada: is pathogen presence a reflection of global and local rat population structure? Vector Borne Zoonotic Dis. 2015;15:21–6. DOIPubMed
- Himsworth CG, Miller RR, Montoya V, Hoang L, Romney MG, Al-Rawahi GN, et al. Carriage of methicillin-resistant Staphylococcus aureus by wild urban Norway rats (Rattus norvegicus). PLoS One. 2014;9:e87983–9. DOIPubMed
No hay comentarios:
Publicar un comentario